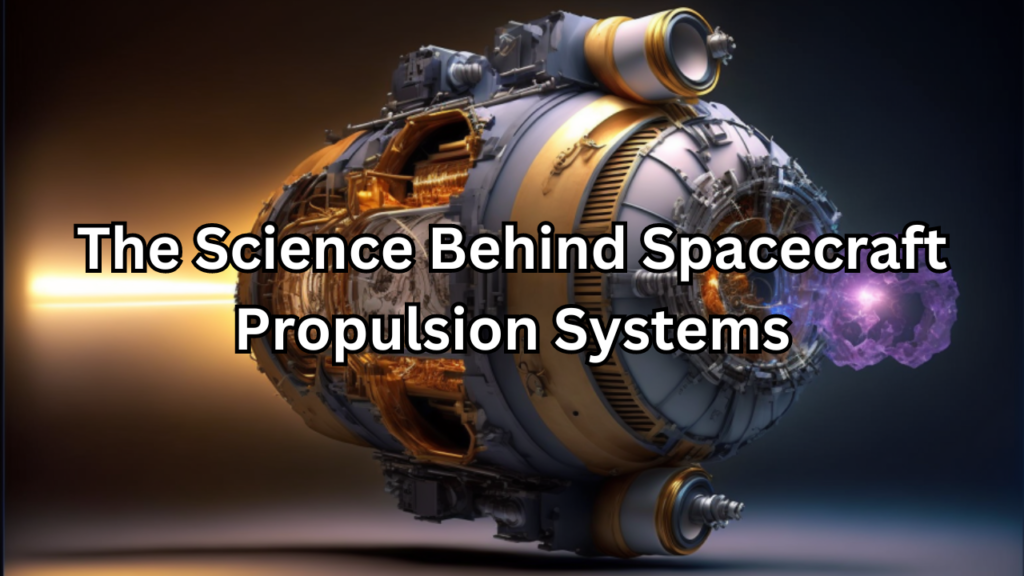
Table of Contents
Introduction
Spacecraft propulsion systems are fundamental to space exploration, providing the means for spacecraft to travel and maneuver through the cosmos. By converting various forms of energy into thrust, these systems allow spacecraft to achieve the velocities and trajectories necessary for reaching and exploring distant celestial bodies. Understanding the science behind these propulsion systems is crucial for advancing space missions and developing new technologies that could significantly impact future exploration endeavors.
Importance of Spacecraft Propulsion Systems
Spacecraft propulsion systems are essential for a variety of reasons. They dictate the range, speed, and maneuverability of spacecraft, influencing mission capabilities and operational efficiency. High-efficiency propulsion systems help reduce fuel consumption and lower mission costs, while precise trajectory control is vital for successful orbital insertion and celestial rendezvous. Technological advancements in propulsion drive innovation across multiple fields, and efficient propulsion is key to conducting ambitious scientific research and expanding our knowledge of the universe.
Brief History of Space Propulsion
The history of space propulsion is marked by significant milestones and innovations. Early 20th-century pioneers like Konstantin Tsiolkovsky and Robert H. Goddard laid the theoretical groundwork for space travel with their concepts of rocket propulsion. The development of the V-2 rocket during World War II demonstrated the potential of rocket technology, leading to rapid advancements during the Space Race era with milestones such as the launch of Sputnik 1 and the Apollo moon landings. The latter half of the 20th century saw the advent of electric propulsion technologies, which revolutionized deep-space missions. Today, ongoing research into advanced propulsion systems promises to further expand the horizons of space exploration.
Basics of Propulsion
Definition and Principles of Propulsion
Propulsion is the mechanism by which spacecraft generate thrust to achieve movement through space. This process involves converting energy into mechanical force to overcome inertia and control motion. In space propulsion, thrust is created by expelling propellant, which propels the spacecraft in the opposite direction. The efficiency of a propulsion system depends on how effectively it converts energy into thrust and manages the propellant.
Newton’s Laws of Motion in Propulsion
Newton’s laws of motion are crucial for understanding spacecraft propulsion. The first law states that an object remains in its state of motion unless acted upon by an external force, explaining the need for thrust to change a spacecraft’s velocity. The second law relates force to mass and acceleration, indicating how the amount of thrust affects acceleration. The third law, stating that for every action there is an equal and opposite reaction, underpins the principle of generating thrust by expelling propellant, resulting in movement in the opposite direction.
Types of Propulsion Systems
Chemical Propulsion
Chemical propulsion is a method of generating thrust by reacting chemical propellants to produce high-speed gases that are expelled to create thrust. This type of propulsion is based on the principles of chemical reactions where the energy released from the combustion of fuel and oxidizer is converted into kinetic energy. Chemical propulsion systems are widely used in space exploration due to their high thrust capabilities and reliability, making them suitable for launching spacecraft and performing major orbital maneuvers.
Liquid Propellant Engines
Liquid propellant engines utilize fuel and oxidizer stored in separate tanks, which are combined and burned in a combustion chamber to produce thrust. The liquid state of the propellants allows for precise control over the combustion process, enabling variable thrust levels and better efficiency. These engines are known for their high performance and flexibility, as they can be shut down and restarted, which is advantageous for complex space missions requiring multiple burns.
Solid Propellant Engines
Solid propellant engines use a single, solid mixture of fuel and oxidizer that is ignited to produce thrust. The propellant is contained within a solid casing and burns from the inside out, generating high-pressure gases that are expelled through a nozzle. While solid propellant engines are simpler and more reliable due to fewer moving parts and less complex fueling systems, they lack the thrust control and reusability features of liquid engines. They are commonly used in booster stages and military applications.
Hybrid Propellant Engines
Hybrid propellant engines combine aspects of both liquid and solid propulsion technologies. They use a liquid oxidizer and a solid fuel, where the oxidizer is injected into the combustion chamber containing the solid fuel. This design allows for the control and adjustability of thrust similar to liquid engines, while benefiting from the simplicity and reliability of solid fuel. Hybrid engines offer a balance of performance, control, and safety, making them a versatile option for various space missions and applications.
Electric Propulsion
Electric propulsion refers to a class of propulsion systems that use electrical energy to accelerate propellant to generate thrust. Unlike chemical propulsion, which relies on combustion, electric propulsion systems use electricity to ionize and accelerate propellant, offering higher efficiency and specific impulse. These systems are particularly valuable for deep-space missions where high efficiency and extended operational durations are crucial. Electric propulsion allows spacecraft to achieve greater speeds over long periods, making it ideal for missions requiring prolonged thrust.
Ion Thrusters
Ion thrusters are a type of electric propulsion system that generates thrust by ionizing a propellant, typically xenon gas, and accelerating the ions through an electric field. The accelerated ions are expelled at high velocities, creating thrust in the opposite direction. Ion thrusters are known for their high specific impulse, meaning they use propellant more efficiently compared to chemical rockets. While they provide low thrust levels, their efficiency makes them suitable for missions requiring long-duration thrust, such as deep-space exploration and satellite station-keeping.
Hall Effect Thrusters
Hall Effect thrusters are another form of electric propulsion that operates by using a magnetic field to ionize and accelerate the propellant. In these thrusters, an electric field created between an anode and a cathode accelerates ions, which are then expelled by the magnetic field. This process provides a higher thrust compared to ion thrusters, making Hall Effect thrusters suitable for various space missions, including satellite propulsion and orbital maneuvers. Their ability to provide a balance between thrust and efficiency makes them a popular choice in current space missions.
Magnetoplasmadynamic (MPD) Thrusters
Magnetoplasmadynamic (MPD) thrusters use magnetic fields to accelerate a plasma (a hot, ionized gas) to generate thrust. These thrusters operate by applying a magnetic field to a plasma created from the propellant, which is then accelerated through an electric field. MPD thrusters offer high thrust capabilities and can operate efficiently over a wide range of power levels. They are considered promising for future deep-space missions and interplanetary travel due to their potential for high performance and efficient energy use. However, challenges remain in optimizing their design and performance for practical space applications.
Nuclear Propulsion
Nuclear propulsion involves using nuclear reactions to generate thrust for spacecraft. This technology harnesses the energy released from nuclear reactions, either through fission or fusion, to provide high levels of thrust and efficiency. Nuclear propulsion offers significant advantages over conventional chemical propulsion, such as higher specific impulse and longer mission durations. It has the potential to enable more ambitious space missions, including deep-space exploration and crewed missions to distant planets. However, nuclear propulsion also presents challenges, including handling radioactive materials and ensuring safety during launch and operation.
Nuclear Thermal Propulsion
Nuclear thermal propulsion (NTP) systems generate thrust by heating a propellant, typically hydrogen, using a nuclear reactor. The heated propellant is then expelled through a nozzle to produce thrust. NTP systems offer a high specific impulse, meaning they are highly efficient in using propellant compared to chemical rockets. This efficiency allows spacecraft to achieve higher speeds and reduce travel times for interplanetary missions. While NTP provides significant performance benefits, it also requires careful management of reactor safety and thermal control to prevent overheating and radiation hazards.
Nuclear Electric Propulsion
Nuclear electric propulsion (NEP) combines nuclear reactors with electric propulsion systems. In NEP, a nuclear reactor generates electricity, which is then used to power electric propulsion systems, such as ion or Hall Effect thrusters. This approach offers the advantage of high efficiency in converting nuclear energy into electrical power and subsequently into thrust. NEP systems are well-suited for long-duration missions, as they can provide continuous thrust over extended periods. However, NEP requires complex systems to manage reactor safety and power distribution, as well as efficient thermal management to handle the heat generated by the reactor.
Chemical Propulsion Systems
Components of Chemical Propulsion
Chemical propulsion systems consist of several key components that work together to produce thrust. The primary components include the propellant tanks, which store the fuel and oxidizer; the combustion chamber, where the chemical reaction occurs; the nozzle, which directs and accelerates the exhaust gases; and the ignition system, which initiates the combustion process. The propellants—fuel and oxidizer—are combined in the combustion chamber, where they react to produce high-pressure gases. These gases are then expelled through the nozzle to create thrust, propelling the spacecraft forward.
Working Mechanism
The working mechanism of chemical propulsion involves a series of steps that convert chemical energy into mechanical thrust. First, the fuel and oxidizer are pumped from their respective tanks into the combustion chamber. In the chamber, the propellants are ignited, resulting in a rapid chemical reaction that produces high-temperature, high-pressure gases. These gases expand and are expelled through the nozzle, generating thrust according to Newton’s third law of motion. The nozzle shape helps to accelerate the gases to high speeds, maximizing the thrust produced and enabling the spacecraft to achieve the desired trajectory or velocity.
Advantages and Disadvantages
Chemical propulsion systems offer several advantages, including high thrust capabilities, simplicity, and proven reliability. Their high thrust is particularly useful for launching spacecraft from Earth’s surface and performing major orbital maneuvers. Chemical propulsion also benefits from a long history of successful use in space missions, contributing to its reliability. However, there are also disadvantages to chemical propulsion. One major drawback is its relatively low specific impulse, meaning it is less efficient in terms of propellant usage compared to other propulsion methods. Additionally, chemical propulsion systems typically have limited reusability and require substantial amounts of propellant, which can make missions more costly. The combustion process also generates high temperatures and pressures, which impose significant engineering challenges related to heat management and material durability.
Electric Propulsion Systems
Principles of Electric Propulsion
Electric propulsion operates on the principle of using electrical energy to accelerate propellant to generate thrust. Unlike chemical propulsion, which relies on the combustion of fuel and oxidizer, electric propulsion systems use electricity to ionize a propellant, such as xenon, and accelerate the ions through electric and magnetic fields. This process involves two key steps: ionization, where the propellant is electrically charged, and acceleration, where the ions are propelled to high velocities. The expelled ions produce thrust in the opposite direction, following Newton’s third law of motion. Electric propulsion is characterized by its high efficiency and specific impulse, making it suitable for long-duration space missions.
Comparison with Chemical Propulsion
Electric propulsion and chemical propulsion differ significantly in terms of performance, efficiency, and application. Chemical propulsion systems provide high thrust, making them ideal for launching spacecraft from Earth and performing major orbital maneuvers. However, they have a lower specific impulse and consume more propellant, which limits their efficiency over long durations. In contrast, electric propulsion systems offer much higher specific impulse, meaning they use propellant more efficiently and can sustain thrust over extended periods. While electric propulsion provides lower thrust compared to chemical systems, its high efficiency makes it suitable for deep-space missions where long-term operation is essential. The choice between the two depends on mission requirements, with chemical propulsion being favored for high-thrust needs and electric propulsion for extended missions.
Applications in Space Missions
Electric propulsion is increasingly used in various space missions due to its efficiency and long-term operational capabilities. Common applications include satellite station-keeping, where electric propulsion systems help maintain a satellite’s position in orbit by making small, precise adjustments. It is also used in deep-space missions, where the high specific impulse allows spacecraft to travel long distances with minimal propellant. Notable examples include the use of ion thrusters on NASA’s Dawn spacecraft for exploring the asteroid belt and the Hall Effect thrusters on several commercial communications satellites. As technology advances, electric propulsion is expected to play a crucial role in future interplanetary missions, enabling more ambitious exploration and extended space travel.
Nuclear Propulsion Systems
Fundamentals of Nuclear Propulsion
Nuclear propulsion utilizes nuclear reactions to generate thrust for spacecraft, leveraging the immense energy released from nuclear fission or fusion. In fission-based systems, the nucleus of heavy elements such as uranium or plutonium is split into smaller nuclei, releasing a large amount of energy that is converted into thermal energy. In fusion-based systems, lighter elements, such as hydrogen isotopes, are fused together at high temperatures, releasing energy. This energy is used to heat a propellant or generate electricity for propulsion systems. Nuclear propulsion offers high efficiency and specific impulse, making it ideal for long-duration missions and deep-space exploration, where conventional propulsion systems may be less effective.
Types and Mechanisms
Nuclear propulsion systems generally fall into two categories: nuclear thermal propulsion (NTP) and nuclear electric propulsion (NEP).
- Nuclear Thermal Propulsion (NTP) involves a nuclear reactor that heats a propellant, such as hydrogen, to high temperatures. The heated propellant is then expelled through a nozzle to produce thrust. This method provides high thrust and specific impulse, making it suitable for missions requiring rapid acceleration and high performance.
- Nuclear Electric Propulsion (NEP) uses a nuclear reactor to generate electricity, which powers electric propulsion systems like ion or Hall Effect thrusters. This approach provides continuous, efficient thrust over long durations, making it ideal for deep-space missions. NEP systems can operate for extended periods, offering high efficiency and lower propellant consumption.
Safety Concerns and Challenges
Nuclear propulsion presents several safety concerns and challenges. One major concern is the handling and containment of radioactive materials. The potential for radioactive contamination during launch, operation, and disposal requires robust safety measures and regulatory oversight. Additionally, the high temperatures and pressures involved in nuclear reactions pose engineering challenges, including thermal management and reactor durability. Another challenge is the complexity of integrating nuclear propulsion systems with spacecraft. Ensuring that the reactor remains stable and secure throughout the mission requires advanced engineering and safety protocols. There are also concerns about the potential for accidents or malfunctions, which could result in the release of radioactive materials. Addressing these concerns involves rigorous testing, safety analysis, and adherence to stringent safety standards to minimize risks and ensure mission success.
Advanced Propulsion Concepts
Solar Sails
Solar sails are a type of propulsion system that uses the pressure of sunlight to propel a spacecraft through space. They consist of large, lightweight, and reflective sails made from materials such as Mylar or Kapton. These sails capture the momentum of photons from the Sun, which exert pressure on the sail’s surface. As the photons strike the sail, they impart a small but continuous force that gradually accelerates the spacecraft. Solar sails offer a unique approach to propulsion by harnessing the constant energy of sunlight, which provides thrust without the need for conventional fuel.
How Solar Sails Work
Solar sails work on the principle of radiation pressure. When sunlight strikes the reflective surface of the sail, photons transfer their momentum to the sail, creating a force that propels the spacecraft forward. The force generated by sunlight is relatively small, but it is continuous and increases with time, allowing the spacecraft to accelerate gradually. The orientation of the sail can be adjusted to control the direction of thrust, enabling the spacecraft to change its trajectory or maintain a stable course. Solar sails require no onboard fuel, as the propulsion relies solely on the energy from the Sun, making them highly efficient for long-duration missions.
Advantages and Potential Applications
Solar sails offer several advantages, including their fuel-free operation, which significantly reduces mission costs and complexity. Their continuous thrust, though small, allows for efficient long-term acceleration, making them well-suited for deep-space missions and interplanetary travel. Solar sails can achieve high speeds over time, enabling exploration of distant celestial bodies with minimal propellant. Potential applications include long-term space missions to explore asteroids, comets, and outer planets, as well as potential use in space telescopes and scientific probes. By leveraging the abundant energy of the Sun, solar sails represent a promising technology for future space exploration.
Antimatter Propulsion
Antimatter propulsion is a cutting-edge concept in spacecraft propulsion that leverages the energy released from matter-antimatter annihilation to generate thrust. In this process, antimatter particles are brought into contact with their matter counterparts, resulting in a highly energetic reaction that converts nearly all of the mass into energy. This energy can be harnessed to propel a spacecraft at high speeds, offering the potential for extremely efficient and powerful propulsion. Antimatter propulsion represents a revolutionary approach with the potential to enable rapid interstellar travel and significantly enhance space exploration capabilities.
Theoretical Basis
The theoretical basis for antimatter propulsion lies in Einstein’s mass-energy equivalence principle, expressed by the equation E=mc2E = mc^2E=mc2. When matter and antimatter collide, they annihilate each other, releasing energy according to this equation. This annihilation process is extraordinarily efficient, converting the entire mass of the particles into energy. In theory, antimatter propulsion systems could achieve specific impulses far exceeding those of conventional chemical or even nuclear propulsion systems. However, producing and storing sufficient antimatter, as well as managing the annihilation reactions safely, remains a significant challenge.
Current Research and Future Prospects
Current research in antimatter propulsion focuses on the production, storage, and containment of antimatter, as well as the development of practical propulsion systems. Antimatter is produced in minute quantities in particle accelerators, but scaling up production to levels required for propulsion is a major hurdle. Researchers are also exploring ways to store and handle antimatter safely, as it requires advanced containment technologies to prevent premature annihilation. Future prospects for antimatter propulsion are promising but require substantial advancements in technology. If these challenges can be overcome, antimatter propulsion could revolutionize space travel by enabling faster-than-current missions to other star systems and deep-space exploration. Ongoing research aims to develop the necessary technologies and infrastructure to make antimatter propulsion a viable option, with potential milestones including more efficient production methods, better storage solutions, and preliminary propulsion experiments in space.
Warp Drives and Faster-than-Light Travel
Warp drives are hypothetical propulsion systems that propose faster-than-light (FTL) travel by bending or “warping” space-time itself. Instead of moving a spacecraft through space at superluminal speeds, a warp drive would alter the fabric of space-time to create a bubble that allows the spacecraft to traverse distances faster than light. This concept aims to circumvent the relativistic limitations imposed by Einstein’s theory of relativity, which states that no object can exceed the speed of light in a vacuum. Warp drives represent a revolutionary approach to space travel, potentially enabling interstellar journeys that are currently beyond our reach.
Scientific Theories
The concept of warp drives is primarily grounded in Einstein’s General Theory of Relativity, which describes how mass and energy warp the fabric of space-time. The most well-known theoretical model for a warp drive is the Alcubierre drive, proposed by physicist Miguel Alcubierre in 1994. Alcubierre’s theory suggests that a spacecraft could achieve FTL travel by contracting space in front of it and expanding space behind it, creating a “warp bubble” that moves the spacecraft faster than light relative to the surrounding space. This model relies on exotic forms of matter with negative energy density, which have not yet been observed or created.
Feasibility and Current Understanding
The feasibility of warp drives remains highly speculative and faces significant challenges. One major obstacle is the requirement for exotic matter or energy with negative energy density, which is not known to exist and is not currently producible with existing technology. Additionally, even if warp drives could be theoretically realized, practical issues such as the enormous energy requirements, stability of the warp bubble, and potential causality violations pose substantial hurdles.
Propulsion System Design
Key Design Considerations
Designing a propulsion system involves several key considerations to ensure its effectiveness and reliability. These include the selection of appropriate propulsion technology based on mission requirements, such as thrust, efficiency, and operational duration. Engineers must account for the spacecraft’s mass, the desired velocity, and the mission profile when designing the propulsion system. Additionally, considerations for thermal management, structural integrity, and safety are crucial to handle the forces and stresses during operation. Integration with the spacecraft’s overall design, including power sources and control systems, is essential for optimal performance and mission success.
Propellant Selection
Propellant selection is a critical factor in propulsion system design, influencing performance, efficiency, and mission costs. The choice of propellant depends on the type of propulsion system being used, whether chemical, electric, or nuclear. For chemical propulsion, the selection involves choosing a suitable fuel and oxidizer that provide the desired thrust and specific impulse. In electric propulsion systems, such as ion or Hall Effect thrusters, inert gases like xenon are commonly used due to their properties that facilitate efficient ionization and acceleration. For nuclear propulsion, selecting appropriate materials for fission or fusion reactions and managing their storage and handling are key considerations. The propellant’s characteristics, including density, energy content, and stability, impact the overall performance and efficiency of the propulsion system.
Thrust and Efficiency Calculations
Thrust and efficiency calculations are essential for evaluating the performance of a propulsion system. Thrust is the force generated by expelling propellant and is determined by factors such as the mass flow rate and velocity of the expelled gases. For chemical propulsion systems, thrust can be calculated using the thrust equation F=m˙ve+(Pe−P0)AeF = \dot{m} v_e + (P_e – P_0) A_eF=m˙ve+(Pe−P0)Ae, where m˙\dot{m}m˙ is the mass flow rate, vev_eve is the exhaust velocity, PeP_ePe is the exit pressure, P0P_0P0 is the ambient pressure, and AeA_eAe is the nozzle area. Efficiency is typically measured by specific impulse (IspI_{sp}Isp), which is the thrust produced per unit of propellant flow rate and is calculated as Isp=Fm˙g0I_{sp} = \frac{F}{\dot{m} g_0}Isp=m˙g0F, where g0g_0g0 is the standard gravity. For electric propulsion, efficiency is measured by how effectively electrical energy is converted into thrust, with calculations involving the power input and the thrust produced. Accurate thrust and efficiency calculations ensure that the propulsion system meets mission objectives and operates within desired performance parameters.
Real-World Applications
Earth Orbit Missions
Earth orbit missions are space missions conducted within or around Earth’s orbit. These missions can involve a range of objectives, including satellite deployment, space station operations, and Earth observation. Satellites are launched into various orbits to perform functions such as communication, weather monitoring, and navigation. Space stations, like the International Space Station (ISS), support scientific research and international collaboration in microgravity environments. Earth orbit missions typically require propulsion systems that can handle periodic adjustments and station-keeping maneuvers. The focus is on maintaining stable orbits, efficient use of resources, and ensuring that the spacecraft or satellite performs its intended functions while orbiting Earth.
Deep Space Missions
Deep space missions extend beyond Earth’s orbit into interplanetary or interstellar space. These missions aim to explore other planets, moons, asteroids, and comets, and to gather data on distant celestial objects. Deep space missions often require advanced propulsion systems capable of long-duration travel and high efficiency, such as electric propulsion or nuclear propulsion. Key challenges include the vast distances involved, requiring sustained thrust and precise navigation over extended periods. These missions are designed to gather scientific data, explore new frontiers, and potentially identify targets for future exploration. Notable examples include missions to Mars, the outer planets, and beyond, such as the Voyager and New Horizons missions.
Manned vs. Unmanned Missions
Manned missions involve human spaceflight and require life support systems, habitat modules, and additional safety measures to protect and sustain astronauts. These missions are typically more complex and costly due to the need for life support, crew training, and emergency protocols. Manned missions often focus on scientific research, exploration, and long-term habitation, such as missions to the ISS or plans for crewed missions to Mars.
Current and Future Research
Innovations in Propulsion Technology
Recent innovations in propulsion technology have significantly advanced space exploration capabilities. Key developments include the refinement of electric propulsion systems, such as ion and Hall Effect thrusters, which offer higher efficiency and longer mission durations compared to traditional chemical rockets. Advances in nuclear propulsion, both thermal and electric, promise to enhance deep-space travel by providing higher thrust and efficiency. Additionally, innovations in solar sail technology have demonstrated the potential for fuel-free propulsion using the pressure of sunlight. Researchers are also exploring emerging technologies such as antimatter propulsion and warp drives, which could revolutionize space travel by enabling faster-than-light journeys. These innovations reflect a growing emphasis on improving propulsion efficiency, reducing mission costs, and expanding the scope of space exploration.
Challenges in Propulsion Research
Propulsion research faces several significant challenges that impact the development and implementation of advanced technologies. One major challenge is the production and storage of exotic materials required for certain propulsion systems, such as antimatter or high-energy propellants. Ensuring safety and reliability in these systems is critical, as they often involve high temperatures, pressures, or radiation. Another challenge is the engineering complexity associated with integrating new propulsion technologies into spacecraft, which requires addressing issues related to thermal management, structural integrity, and power systems. Additionally, the high costs and technical difficulties of testing and validating advanced propulsion concepts pose barriers to progress. Overcoming these challenges requires continued investment in research, development, and collaboration across scientific and engineering disciplines.
Future Prospects and Goals
The future of propulsion technology holds exciting prospects as researchers and engineers continue to push the boundaries of space exploration. Key goals include achieving more efficient and powerful propulsion systems to enable faster interplanetary and interstellar travel. Advances in technologies such as nuclear propulsion, solar sails, and potential breakthrough concepts like antimatter and warp drives aim to make long-duration space missions more feasible and cost-effective. Future prospects also include the development of reusable propulsion systems to reduce mission costs and increase the frequency of space travel. Additionally, there is a growing focus on sustainable and environmentally friendly propulsion technologies that minimize the impact on space environments and planetary bodies. Achieving these goals will require continued innovation, interdisciplinary collaboration, and significant advancements in materials science, energy management, and spacecraft design.
Environmental and Ethical Considerations
Impact on Space Environment
The impact of propulsion systems on the space environment is a critical consideration in spacecraft design and operation. Propulsion systems can contribute to space debris, which poses risks to other spacecraft and satellites. Chemical propulsion systems, in particular, can release pollutants or fragments that contribute to the growing problem of space junk. Electric and nuclear propulsion systems, while offering high efficiency and low environmental impact in terms of emissions, still need to address issues such as the potential for radioactive contamination in the case of nuclear propulsion. Ensuring that propulsion technologies minimize their footprint and do not exacerbate space debris is essential for maintaining a sustainable and safe space environment.
Ethical Issues in Nuclear Propulsion
Nuclear propulsion raises several ethical issues related to safety, environmental impact, and the potential for misuse. One major concern is the safe handling and disposal of radioactive materials, which require stringent safety measures to prevent accidents and contamination. The potential for a launch failure or spacecraft malfunction involving nuclear propulsion could have severe environmental and health consequences. Additionally, there are ethical considerations regarding the use of nuclear technology in space exploration, including the potential for the weaponization of nuclear propulsion systems or their use in harmful ways. Ensuring transparency, robust safety protocols, and international cooperation is crucial to address these ethical issues and maintain public trust in space exploration initiatives.
Sustainability in Space Exploration
Sustainability in space exploration involves developing technologies and practices that minimize environmental impact and ensure the long-term viability of space missions. Key aspects of sustainability include the efficient use of resources, reduction of space debris, and the implementation of reusable technologies. Sustainable practices in propulsion technology focus on creating systems that use minimal or non-toxic propellants, reduce waste, and lower the overall environmental footprint. Additionally, there is a growing emphasis on the use of in-situ resources, such as extracting water or other materials from celestial bodies, to support long-term missions and reduce reliance on Earth-based supplies. Promoting sustainability in space exploration is essential for preserving space environments and enabling future generations to continue exploring and utilizing space.
Summary
Spacecraft propulsion is a crucial aspect of space exploration, enabling spacecraft to achieve and maintain their trajectories, whether in Earth’s orbit or traveling to distant celestial bodies. Propulsion systems vary widely, from traditional chemical rockets to advanced electric and nuclear technologies. Each type of propulsion system has unique advantages and limitations, influencing mission design and execution. Innovations such as solar sails, antimatter propulsion, and theoretical concepts like warp drives are pushing the boundaries of what is possible in space travel. Understanding the principles, challenges, and future prospects of propulsion technology is essential for advancing space exploration and addressing the complex needs of future missions.
Recap of Key Points
- Definition and Principles of Propulsion: Propulsion systems operate based on fundamental principles of physics, including Newton’s laws of motion. Various types of propulsion systems utilize different methods to generate thrust, such as chemical reactions, electrical energy, or nuclear reactions.
- Types of Propulsion: Chemical propulsion, including liquid, solid, and hybrid engines, offers high thrust but lower efficiency. Electric propulsion systems, like ion and Hall Effect thrusters, provide high efficiency with lower thrust. Nuclear propulsion, including thermal and electric variants, promises significant advancements for deep-space travel.
- Innovations and Challenges: Recent advancements in propulsion technologies include the development of more efficient electric propulsion systems, solar sails, and research into antimatter and warp drives. However, challenges such as high costs, technical complexity, and safety concerns must be addressed.
- Impact on Space Environment: Propulsion systems can contribute to space debris and environmental risks, necessitating careful management and sustainable practices.
- Ethical and Sustainability Issues: Ethical considerations in propulsion technology, especially concerning nuclear propulsion, include safety and environmental impact. Sustainability in space exploration focuses on resource efficiency, reducing space debris, and developing reusable technologies.
The Future of Spacecraft Propulsion
The future of spacecraft propulsion is poised for transformative developments as researchers and engineers continue to explore new technologies and refine existing systems. Advances in propulsion will likely focus on enhancing efficiency, enabling faster and more cost-effective missions, and expanding the range of space exploration. Innovations such as advanced nuclear propulsion, more efficient electric thrusters, and novel concepts like antimatter and warp drives could revolutionize space travel. The integration of sustainable practices and the development of reusable systems will also play a crucial role in supporting long-term exploration goals. As technology evolves, the potential for groundbreaking discoveries and deeper space exploration will increase, paving the way for new frontiers in our understanding of the universe.
FAQs
What is the most efficient type of propulsion system?
The efficiency of a propulsion system is often measured by its specific impulse, which represents the thrust produced per unit of propellant consumed. Among current technologies, electric propulsion systems, such as ion thrusters and Hall Effect thrusters, are the most efficient in terms of specific impulse. These systems can achieve high velocities with minimal propellant, making them ideal for long-duration space missions. However, they produce lower thrust compared to chemical rockets, which are more suitable for launches and rapid maneuvers.
How do electric propulsion systems differ from chemical ones?
Electric propulsion systems differ from chemical propulsion systems primarily in their method of generating thrust. Electric propulsion uses electrical energy to ionize and accelerate a propellant, such as xenon, to produce thrust. This results in high efficiency and specific impulse but lower thrust levels. In contrast, chemical propulsion systems rely on the combustion of fuel and oxidizer to produce high-thrust, short-duration propulsion. Chemical rockets generate significant thrust and are commonly used for launch and major orbital maneuvers, but they have lower specific impulse and consume more propellant.
What are the main challenges in developing nuclear propulsion?
Developing nuclear propulsion faces several significant challenges. These include:
- Safety: Handling and containing radioactive materials require stringent safety measures to prevent accidents and contamination.
- Engineering Complexity: Designing and integrating nuclear reactors into spacecraft involves complex engineering to manage high temperatures, pressures, and radiation.
- Cost: The development, testing, and implementation of nuclear propulsion systems are costly and require substantial investment.
- Regulation: International regulations and agreements govern the use of nuclear technology in space, adding to the complexity of development and deployment.
Are advanced propulsion concepts like warp drives feasible?
Advanced propulsion concepts such as warp drives remain theoretical and face significant scientific and engineering challenges. Warp drives, which propose to bend space-time to achieve faster-than-light travel, rely on hypothetical exotic matter with negative energy density, which has not been observed or created. Current understanding of physics does not support the feasibility of warp drives with existing technology. Research in theoretical physics continues, but practical implementation remains speculative and distant.
What are the environmental impacts of spacecraft propulsion systems?
Spacecraft propulsion systems can have various environmental impacts, including:
- Space Debris: Propulsion systems, particularly chemical rockets, can contribute to space debris, posing risks to other spacecraft and satellites.
- Pollutants: Chemical propulsion systems can release pollutants and fragments into space, which may contribute to the growing problem of space junk.
- Radioactive Contamination: Nuclear propulsion systems pose risks of radioactive contamination if there are accidents or failures.
- Sustainability: The environmental footprint of propulsion technologies, including resource use and waste production, impacts the long-term sustainability of space exploration.