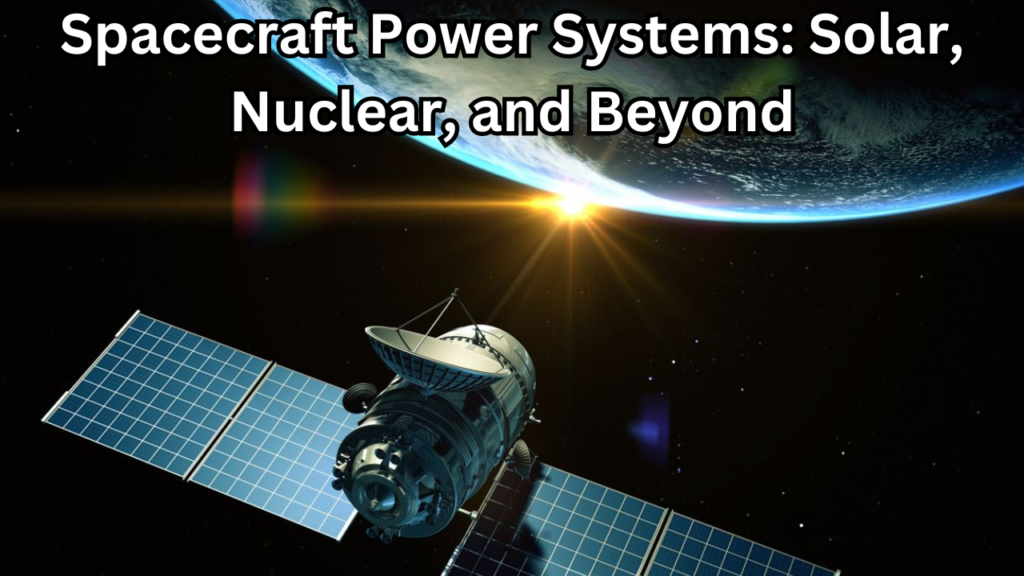
Table of Contents
Introduction
Overview of Spacecraft Power Systems
Spacecraft power systems are integral to the functioning of modern space missions. These systems provide the necessary energy for all spacecraft operations, from propulsion and communications to scientific experiments and onboard systems. The challenges of operating in space—such as the absence of conventional power sources and extreme environmental conditions—necessitate highly reliable and efficient power systems.
There are various types of power systems used in spacecraft, each designed to meet specific mission requirements. These include solar power systems, which harness sunlight to generate electricity; nuclear power systems, which utilize radioactive decay or nuclear reactions; and emerging technologies that promise to enhance or supplement existing power sources. Each power system has its own set of advantages and limitations, influencing its suitability for different types of missions.
Understanding spacecraft power systems involves exploring the principles behind these technologies, their historical development, and the advancements that continue to shape their evolution. As space missions become more ambitious, the role of power systems becomes increasingly crucial in ensuring mission success and longevity.
Importance of Reliable Power in Space Exploration
In the realm of space exploration, where conventional power infrastructure is absent, the reliability of power systems is of paramount importance. Spacecraft operate in an environment where power must be generated, stored, and managed independently. A failure in the power system can result in the loss of critical data, mission objectives, or even the spacecraft itself.
Reliable power is essential for several reasons:
- Sustaining Operations: Spacecraft rely on power for propulsion systems, communication with mission control, scientific instruments, and life support systems (in the case of crewed missions). Any interruption in power can jeopardize these operations.
- Long-Duration Missions: Missions that extend beyond Earth’s orbit, such as those to Mars or outer planets, require power systems that can function reliably over extended periods. Solar power systems might become less effective far from the Sun, while nuclear power systems must provide consistent energy throughout the mission.
- Mission Safety and Success: A dependable power source ensures that spacecraft can execute planned activities and respond to unforeseen challenges. It contributes to the overall safety of the mission by minimizing the risk of power-related failures.
- Scientific Research and Data Collection: Space missions often aim to gather valuable scientific data, which requires continuous operation of onboard instruments. Reliable power systems are essential for maintaining these instruments and ensuring accurate data collection.
In summary, the reliability of power systems is a critical factor that influences the success and safety of space missions. As space exploration continues to advance, the development and refinement of power systems remain a key focus to support increasingly complex and distant missions.
Solar Power Systems
Basics of Solar Power
How Solar Panels Work
Solar panels operate on the principle of converting sunlight into electricity through the photovoltaic effect. The photovoltaic effect occurs when light photons hit a semiconductor material, such as silicon, in the solar cells of the panel. This energy excites electrons within the semiconductor, creating an electric current. The flow of electrons is captured and directed through a circuit to produce usable electricity.
Solar panels consist of multiple photovoltaic cells connected together. Each cell contains a positive and a negative layer of semiconductor material, creating an electric field. When photons from sunlight strike the cells, they release electrons, which are then collected by the electric field and flow through the external circuit, generating direct current (DC) electricity. This DC electricity can be converted into alternating current (AC) by an inverter for use in various applications.
Historical Development of Solar Power in Space
The use of solar power in space exploration began with early space missions, reflecting the need for reliable and efficient energy sources in the vacuum of space. The first solar-powered spacecraft were launched in the 1960s, showcasing the potential of solar panels for generating electricity in space.
The advancement of solar technology for space applications has been driven by the need to provide power for a range of missions, from low Earth orbit satellites to deep space probes. Over the decades, solar panels have become increasingly efficient, lightweight, and capable of functioning in the harsh conditions in space.
In the early missions, solar panels were relatively bulky and less efficient. However, technological progress has led to significant improvements in solar cell efficiency and panel design, allowing spacecraft to utilize solar power more effectively. The development of high-efficiency cells and advanced materials has expanded the possibilities for solar-powered space missions.
Types of Solar Panels
Monocrystalline Solar Panels
Monocrystalline solar panels are made from a single continuous crystal structure. They are known for their high efficiency and longevity. These panels are typically black and have a uniform appearance due to the single-crystal silicon used in their production. The efficiency of monocrystalline panels can exceed 20%, making them one of the most effective types of solar panels available.
Polycrystalline Solar Panels
Polycrystalline solar panels are made from multiple silicon crystals melted together. These panels have a blueish hue and are less expensive to manufacture than monocrystalline panels. Although they are slightly less efficient (usually around 15-17%), they offer a good balance between cost and performance. Polycrystalline panels are commonly used in various applications, including space missions, where cost-effectiveness is important.
Thin-Film Solar Panels
Thin-film solar panels are made from layers of photovoltaic material deposited onto a substrate. These panels are lightweight and flexible, which makes them suitable for applications where weight and space are constraints. However, their efficiency is generally lower compared to crystalline panels, typically ranging from 10-12%. Thin-film panels are used in space missions where their flexibility and light weight can be advantageous, despite their lower efficiency.
Advancements in Solar Technology
High-Efficiency Solar Cells
Recent advancements in solar technology have led to the development of high-efficiency solar cells. These cells utilize new materials and manufacturing techniques to achieve efficiency rates above 25%. Innovations such as multi-junction cells, which layer multiple semiconductor materials to capture a broader spectrum of sunlight, have significantly improved performance. These high-efficiency cells are essential for space missions, where maximizing power generation is crucial.
Lightweight and Flexible Panels
The development of lightweight and flexible solar panels has revolutionized their use in space exploration. Advances in materials science have led to the creation of panels that can be rolled or folded, reducing the space required for deployment. These panels are particularly useful for spacecrafts with limited storage or launch volume, as they can be expanded once in orbit to maximize energy capture.
Solar Power for Deep Space Missions
Solar power for deep space missions presents unique challenges due to the decreased intensity of sunlight at greater distances from the Sun. To address this, engineers have developed advanced solar arrays with larger surface areas and improved efficiency. Techniques such as high-efficiency cells and lightweight structures help ensure that spacecraft can generate sufficient power even in the outer reaches of the solar system.
Case Studies
Solar-Powered Spacecraft: Past and Present
Solar power has been a cornerstone of space exploration since the early days of satellite technology. Early missions, such as the first artificial satellites, relied on solar panels to provide power in space. Over time, the technology has evolved to support more complex and demanding missions. Modern solar-powered spacecraft, such as the International Space Station (ISS) and various scientific probes, continue to rely on solar energy for their operations.
Success Stories: Juno, Voyager, and More
The Juno spacecraft, launched by NASA to study Jupiter, is a notable example of a modern solar-powered mission. Despite being far from the Sun, Juno utilizes advanced solar arrays to power its instruments and communication systems. The Voyager probes, launched in the 1970s, also relied on solar panels for their initial power needs, showcasing the effectiveness of solar energy for interplanetary travel. These success stories highlight the ongoing relevance and evolution of solar power in space exploration, demonstrating its capability to support missions across different environments and distances from the Sun.
Nuclear Power Systems
Fundamentals of Nuclear Power
Nuclear power is a method of generating energy through nuclear reactions. The primary types of nuclear power used in spacecraft are Radioisotope Thermoelectric Generators (RTGs) and nuclear fission reactors. Each method harnesses the energy released from nuclear processes to produce electricity.
Radioisotope Thermoelectric Generators (RTGs)
RTGs are devices that convert the heat released by the natural radioactive decay of certain isotopes into electricity. This process involves using thermoelectric materials that generate electrical power from temperature differences. RTGs are commonly used in space missions where long-term, reliable power is needed without reliance on solar energy.
How RTGs Work
RTGs consist of a radioactive material, such as plutonium-238, which emits heat as it decays. This heat is transferred to thermoelectric generators, which convert the thermal energy into electrical power. The electricity produced is used to power the spacecraft’s instruments and systems. RTGs have been used in missions such as the Voyager and Curiosity rovers.
Nuclear Fission Reactors
Nuclear fission reactors generate power by splitting heavy atomic nuclei, such as uranium or plutonium, into lighter nuclei. This process releases a significant amount of energy, which can be used to generate electricity. Spacecraft equipped with nuclear fission reactors can produce large amounts of power, making them suitable for missions with high energy demands.
How Nuclear Fission Reactors Work
In a nuclear fission reactor, a chain reaction is initiated by bombarding a fissile material with neutrons. The splitting of atomic nuclei releases energy in the form of heat. This heat is then used to produce steam, which drives a turbine connected to a generator that produces electricity. Reactors are designed with extensive safety measures to control the reaction and manage the heat produced.
Benefits of Nuclear Power
Longevity and Reliability
Nuclear power systems offer exceptional longevity and reliability. RTGs can function for several decades without the need for refueling or maintenance, making them ideal for long-duration space missions. Nuclear reactors also provide a consistent power output over extended periods, which is crucial for deep space missions where solar power might be insufficient.
High Energy Density
Nuclear power provides a high energy density compared to other power sources. The energy released from nuclear reactions is orders of magnitude greater than that from chemical or solar sources. This high energy density allows spacecraft to operate with smaller fuel volumes, reducing weight and storage requirements.
Safety and Efficiency
Radiation Shielding and Safety Measures
Safety is a critical consideration in nuclear power systems, particularly in space where radiation exposure is a concern. Advanced radiation shielding is used to protect spacecraft components and crew from harmful radiation. Materials such as lead and borated polyethylene are employed to absorb and block radiation, ensuring the safety of both the spacecraft and its instruments.
Advances in Nuclear Technology
Recent advances in nuclear technology focus on improving the safety, efficiency, and performance of power systems. Innovations include more robust reactor designs, enhanced radiation shielding materials, and better waste management strategies. These advancements aim to address challenges related to nuclear power while expanding its applications in space exploration.
Case Studies
Notable Nuclear-Powered Missions: Cassini, Curiosity, and More
Several significant space missions have utilized nuclear power systems to achieve their objectives:
- Cassini: Launched in 1997, the Cassini spacecraft used an RTG to explore Saturn and its moons. The RTG provided reliable power for its scientific instruments and communication systems throughout its mission, which lasted more than 13 years.
- Curiosity: The Mars rover Curiosity, launched in 2011, is powered by an RTG. The nuclear power system has enabled Curiosity to conduct extensive scientific research on Mars, providing a continuous power supply regardless of the planet’s sunlight conditions.
- Voyager Probes: The Voyager 1 and Voyager 2 spacecraft, launched in 1977, are powered by RTGs. These probes have traveled beyond the solar system, continuing to send valuable data back to Earth thanks to their reliable nuclear power sources.
Future Prospects: NASA’s Kilopower Project
NASA’s Kilopower project aims to develop compact nuclear reactors for future space missions. The Kilopower reactor is designed to provide a reliable and efficient power source for long-duration missions on the Moon, Mars, and beyond. It features a small, lightweight design with advanced safety and efficiency features. The Kilopower project represents a significant step toward expanding the use of nuclear power in space exploration, addressing the need for sustainable and robust power systems for future missions.
Alternative Power Systems
Chemical Power Systems
Chemical power systems utilize chemical reactions to generate electrical energy or thrust for spacecraft. These systems are integral for various functions in space missions, including power supply and propulsion.
Batteries and Fuel Cells
Batteries
Batteries store electrical energy in chemical form and release it as needed. They consist of electrochemical cells that convert chemical energy into electrical energy. Different types of batteries are used in spacecraft depending on their specific needs:
- Lithium-Ion Batteries: These batteries are popular in space missions due to their high energy density, light weight, and long life cycle. They are used in many modern spacecraft, including satellites and space probes.
- Nickel-Hydrogen Batteries: Known for their durability and resistance to radiation, nickel-hydrogen batteries are commonly used in long-duration space missions. They can withstand harsh conditions and provide reliable power over extended periods.
Fuel Cells
Fuel cells generate electricity through chemical reactions between a fuel and an oxidizer. In space applications, fuel cells are often used to provide a clean, efficient power source:
- Hydrogen Fuel Cells: These cells convert hydrogen and oxygen into electricity, producing water as a byproduct. They offer high efficiency and are used in various spacecraft, including the Space Shuttle’s fuel cell systems.
- Regenerative Fuel Cells: These cells use excess electrical energy to split water into hydrogen and oxygen, which can later be recombined to produce electricity. They offer the advantage of being able to store energy in the form of fuel.
Chemical Propulsion
Chemical propulsion systems use the energy from chemical reactions to produce thrust, which is essential for maneuvering and controlling spacecraft:
- Liquid Rocket Engines: These engines use liquid propellants, such as liquid hydrogen and liquid oxygen, which are mixed and burned in a combustion chamber to generate high-speed exhaust gases. Liquid engines offer precise control over thrust and are used in many launch vehicles.
- Solid Rocket Motors: Solid propellants are pre-loaded into a chamber and ignited to produce thrust. While simpler and more reliable than liquid engines, solid motors lack the same level of thrust control.
Emerging Technologies
Dynamic Power Conversion
Dynamic power conversion involves technologies that efficiently convert electrical power between different forms or voltages based on real-time needs:
- Advanced DC-DC Converters: These converters are designed to handle varying voltage levels and optimize power distribution. They are crucial for managing the power needs of different spacecraft systems and components.
- Adaptive Power Management Systems: These systems dynamically adjust power usage and distribution according to mission requirements, enhancing overall efficiency and reducing waste.
Wireless Power Transmission
Wireless power transmission (WPT) allows for the transfer of electrical energy without physical connections. This technology is being explored for its potential benefits in space:
- Microwave Power Transmission: This method involves converting electrical energy into microwave beams that can be transmitted through space and converted back into electricity at the receiving end. It offers a potential solution for powering spacecraft or beaming energy from space-based solar power stations.
- Resonant Inductive Coupling: WPT using resonant inductive coupling involves transferring power between coils tuned to the same resonant frequency. This technology could be used for charging spacecraft or equipment without direct contact.
Innovative Concepts
Space-Based Solar Power Stations
Space-based solar power stations are futuristic concepts that involve deploying solar panels in orbit to capture solar energy continuously and beam it to Earth or other spacecraft:
- Solar Power Satellites: These satellites would collect solar energy using large arrays and convert it into microwave or laser beams for transmission. The energy could be received on Earth or in space and used to provide power to various systems.
- Space Solar Farms: These would consist of extensive arrays of solar panels in orbit, providing a constant supply of power unaffected by Earth’s atmosphere or night cycles.
Antimatter and Fusion Power
Antimatter
Antimatter power involves harnessing the energy released from antimatter-matter annihilation. When antimatter particles interact with matter, they annihilate each other, producing tremendous amounts of energy:
- Antimatter Propulsion: This concept envisions using the energy from antimatter annihilation to propel spacecraft at extremely high speeds. However, practical implementation faces significant challenges, including antimatter production and storage.
Fusion Power
Nuclear fusion is the process of combining light atomic nuclei to form heavier nuclei, releasing a vast amount of energy:
- Deuterium-Tritium Fusion: The most studied fusion reaction uses isotopes of hydrogen to produce helium and energy. Experimental reactors like ITER are working toward achieving controlled fusion for power generation.
- Compact Fusion Reactors: Researchers are exploring smaller fusion reactors that could potentially provide power for spacecraft. These reactors aim to make fusion a practical energy source for space missions.
Comparison of Power Systems
Efficiency and Output
Efficiency and output are crucial metrics for evaluating power systems used in space missions. Efficiency refers to how well a power system converts its energy source into usable electrical power, while output measures the total amount of power produced. Solar power systems, such as photovoltaic panels, have high efficiency rates but can vary based on the intensity of sunlight, which affects their output. In contrast, nuclear power systems, including Radioisotope Thermoelectric Generators (RTGs) and fission reactors, generally offer higher and more consistent output regardless of environmental conditions. The choice between solar and nuclear power often hinges on the specific energy requirements of the mission and the reliability needed for its duration.
Solar vs. Nuclear: Performance Metrics
When comparing solar and nuclear power systems, performance metrics such as energy density, longevity, and reliability play significant roles. Solar panels offer the advantage of being lightweight and well-suited for environments with consistent sunlight, but their performance can be affected by factors such as distance from the sun and the duration of eclipses. Nuclear power systems, on the other hand, provide a high energy density and are less dependent on external environmental conditions, making them ideal for missions in deep space or where prolonged energy supply is crucial. While solar panels require regular maintenance and alignment, nuclear systems, particularly RTGs, offer a long-term power solution with minimal maintenance.
Adaptability to Different Missions
The adaptability of power systems to different missions is a critical factor in their selection. Solar power systems are highly adaptable for missions within close proximity to the Sun, such as those in low Earth orbit or around the inner planets, where sunlight is plentiful. They can be adjusted for varying energy needs by changing the orientation of solar panels. Conversely, nuclear power systems are well-suited for missions that venture into deeper space or require consistent power over long durations, as they are less influenced by the distance from the Sun and can provide a steady energy supply. The choice of power system often depends on the specific requirements of the mission, including its duration, distance from the Sun, and the type of payload.
Cost and Sustainability
Cost and sustainability are important considerations when evaluating power systems for space missions. Solar power systems generally have lower initial costs compared to nuclear systems, due to simpler technology and manufacturing processes. However, their efficiency and effectiveness can be affected by the mission’s location and environmental conditions. Nuclear power systems, while more expensive to develop and launch, offer the benefit of long-term sustainability with fewer maintenance requirements. They provide a reliable energy source with minimal dependence on external factors. The decision often involves a trade-off between the initial investment and the long-term benefits, with sustainability playing a key role in the feasibility of extended space missions.
Economic Considerations
Economic considerations involve evaluating both the upfront costs and the long-term financial implications of different power systems. Solar power systems, although typically less expensive to produce and deploy, may require additional resources for maintenance and adjustments, particularly for missions extending beyond the solar system. Nuclear power systems entail higher initial costs due to the complexity of the technology and stringent safety measures but offer significant advantages in terms of longevity and reliability. The total cost of a space mission must account for the power system’s performance over its expected lifetime, including potential savings from reduced maintenance and increased mission duration.
Environmental Impact
The environmental impact of power systems is an important factor in their selection. Solar power systems are generally considered environmentally friendly as they harness energy from the Sun without producing harmful emissions or waste. They rely on renewable energy and have minimal impact on the space environment. Nuclear power systems, while offering high efficiency and reliability, involve considerations related to radioactive materials and waste. The production and handling of nuclear fuel and the potential for radioactive contamination must be carefully managed to mitigate environmental risks. Both power systems require thoughtful consideration of their impact on space missions and the broader space environment.
Mission Suitability
The suitability of power systems for specific missions depends on their ability to meet the mission’s energy requirements and operational conditions. Solar power systems are well-suited for missions within the inner solar system, where sunlight is abundant, and their performance can be enhanced by optimal panel positioning. In contrast, nuclear power systems are preferred for missions to the outer planets or deep space, where solar energy is insufficient and a reliable, long-term power source is needed. Each power system’s characteristics must align with the mission’s objectives, duration, and environmental conditions to ensure successful operation.
Short-Term vs. Long-Term Missions
The choice between power systems for short-term and long-term missions is influenced by their respective advantages. For short-term missions, such as those in low Earth orbit or around nearby planets, solar power systems may be sufficient and cost-effective due to their lower initial expense and adequate performance within the Sun’s reach. For long-term missions, such as those exploring distant planets or traveling beyond the solar system, nuclear power systems are often preferred due to their consistent output and longevity. The decision is driven by the mission’s duration, energy needs, and the expected operational environment.
Manned vs. Unmanned Missions
The type of mission—manned or unmanned—affects the choice of power systems. Manned missions require highly reliable and safe power sources, as any power failure can directly impact the safety and success of the crew. Nuclear power systems, with their long-term reliability and high energy density, are often chosen for crewed missions in deep space. Unmanned missions, while also benefiting from reliable power sources, have more flexibility in their power system choice. Solar power systems can be sufficient for many unmanned missions, especially those within the inner solar system, where the cost and complexity of nuclear systems may not be justified. The decision depends on balancing reliability, safety, and cost against the mission’s specific requirements.
Challenges and Future Directions
Technical Challenges
Technical challenges in space power systems encompass a range of issues that impact the effectiveness and reliability of power sources. One significant challenge is ensuring power systems operate efficiently in the extreme conditions of space, including temperature fluctuations, radiation, and vacuum. Solar panels, for instance, must be able to withstand intense solar radiation and thermal extremes while maintaining their efficiency. Similarly, nuclear power systems require advanced shielding to protect against radiation and manage heat dissipation effectively. Additionally, integrating power systems with spacecraft technology poses challenges related to weight, volume, and the need for robust, fail-safe mechanisms to ensure uninterrupted operation.
Durability in Space Conditions
Durability in space conditions is crucial for the longevity and performance of power systems. Spacecraft operate in a harsh environment where components are exposed to intense radiation, micrometeoroids, and extreme temperatures. Solar panels must be designed to endure high-energy particles and thermal stresses without degrading. Coatings and materials that can withstand radiation and thermal cycling are essential for maintaining efficiency over time. Nuclear power systems also face durability challenges, as they must be able to operate reliably over extended periods while managing radioactive decay and thermal output. Ensuring durability involves rigorous testing and the use of advanced materials designed to withstand the rigors of space.
Integration with Spacecraft Design
Integrating power systems with spacecraft design is a complex process that involves balancing power needs with spacecraft constraints. Power systems must be incorporated into the spacecraft’s design without compromising structural integrity, aerodynamic properties, or mission payloads. Solar panels need to be mounted in positions that optimize sunlight exposure while accommodating the spacecraft’s operational maneuvers. Nuclear power systems, with their associated shielding and heat management requirements, must be carefully integrated to ensure safety and functionality. This integration often involves close collaboration between power system engineers and spacecraft designers to achieve optimal performance and reliability.
Research and Development
Research and development (R&D) play a critical role in advancing space power systems. R&D efforts focus on improving the efficiency, reliability, and safety of existing technologies while exploring new approaches. For solar power, R&D might involve developing higher-efficiency photovoltaic materials or innovative panel designs. In the field of nuclear power, research is directed at enhancing reactor designs, safety protocols, and waste management solutions. The development of new power technologies also includes exploring cutting-edge concepts such as advanced energy storage solutions and novel propulsion methods. Continuous R&D is essential for addressing the evolving demands of space missions and pushing the boundaries of what is possible.
Cutting-Edge Research Projects
Cutting-edge research projects are driving the future of space power systems by exploring innovative technologies and approaches. Projects like NASA’s Kilopower, which aims to develop compact nuclear reactors for space missions, represent significant advancements in power generation. Similarly, research into space-based solar power stations seeks to harness solar energy from orbit and transmit it to Earth or other spacecraft. These projects often involve collaboration between space agencies, research institutions, and private industry to push the envelope of current technology and address the unique challenges of space environments.
Collaborative Efforts in Space Power Systems
Collaborative efforts in space power systems are essential for advancing technology and overcoming the complex challenges of space exploration. International partnerships between space agencies, such as NASA, ESA, and Roscosmos, often pool resources and expertise to develop and deploy advanced power systems. Collaborative projects may involve joint research initiatives, shared funding, and coordinated development efforts. These partnerships enable the pooling of knowledge, resources, and technology to tackle the significant engineering and scientific challenges associated with space power systems, resulting in more robust and innovative solutions.
Future Trends
Future trends in space power systems are likely to be shaped by advancements in technology and the evolving needs of space missions. Emerging trends include the development of more efficient and lightweight power generation systems, such as next-generation solar panels and compact nuclear reactors. There is also growing interest in exploring alternative power sources, such as antimatter and fusion power, which could revolutionize space travel with their potential for high energy density. Additionally, innovations in energy storage and management, including advanced battery technologies and wireless power transfer, will play a crucial role in enhancing the performance and reliability of space power systems.
Potential Breakthroughs
Potential breakthroughs in space power systems could significantly impact the future of space exploration. Advances in fusion power, which promise nearly limitless and clean energy, could transform the way spacecraft are powered and enable longer and more ambitious missions. Breakthroughs in antimatter technology could provide extremely high energy densities, revolutionizing propulsion systems and travel times. Additionally, developments in materials science could lead to the creation of more durable and efficient solar panels and nuclear reactors. These breakthroughs have the potential to address current limitations and open new frontiers in space exploration.
Vision for Future Space Missions
The vision for future space missions includes expanding human presence beyond Earth and exploring deeper into the solar system and beyond. Power systems will play a crucial role in achieving these goals, providing reliable and sustainable energy for a wide range of missions. Future missions may involve establishing permanent lunar bases, sending crewed missions to Mars, and exploring the outer planets and their moons. Advanced power systems will be essential for supporting life support systems, scientific instruments, and propulsion technologies required for these ambitious objectives. As space exploration evolves, the development of innovative and efficient power systems will be key to realizing the vision of a more extensive and interconnected spacefaring future.
Summary
Recap of Key Points
In reviewing the key points regarding spacecraft power systems, several crucial aspects emerge. First, the choice between solar and nuclear power systems is influenced by factors such as efficiency, output, adaptability, and mission duration. Solar power, with its high efficiency and lower initial costs, is well-suited for missions within the inner solar system where sunlight is abundant. However, it faces limitations in deep space where sunlight is weak. Nuclear power systems, including Radioisotope Thermoelectric Generators (RTGs) and fission reactors, offer high energy density and reliability, making them ideal for deep space missions and long-term operations. Technical challenges, including durability in harsh space conditions and integration with spacecraft design, are critical considerations in ensuring the effectiveness of these power systems. Emerging technologies such as dynamic power conversion and wireless power transmission are paving the way for more advanced and flexible power solutions. Innovative concepts, including space-based solar power stations and exploration of antimatter and fusion power, represent the forefront of research and development in space Technology power systems. Collaborative efforts and cutting-edge research projects are driving advancements, and future trends promise significant breakthroughs that could revolutionize space exploration.
The Future of Spacecraft Power Systems
The future of spacecraft power systems is poised for transformative advancements driven by ongoing research and technological innovation. As space missions become more ambitious, including extended missions to Mars, the outer planets, and beyond, the need for reliable, efficient, and sustainable power sources will grow. Advances in solar power technology, such as higher-efficiency cells and flexible panels, will enhance performance in diverse space environments. Meanwhile, developments in nuclear power, including compact fusion reactors and improved RTGs, promise to provide long-term, high-density energy solutions for deep space exploration. Emerging technologies like space-based solar power stations and breakthroughs in antimatter and fusion power could redefine energy generation and propulsion, potentially enabling faster travel and more complex missions. Additionally, the integration of new energy storage solutions and wireless power transmission technologies will enhance the flexibility and efficiency of power systems. As these innovations continue to evolve, they will play a pivotal role in expanding humanity’s reach into space, supporting new scientific discoveries, and establishing a sustainable presence beyond Earth.
FAQs
What is the Most Efficient Power System for Space Missions?
Determining the most efficient power system for space missions depends on the specific mission requirements and environmental conditions. Solar power systems are generally considered highly efficient for missions within the inner solar system where sunlight is abundant. Modern photovoltaic panels can convert a significant portion of solar energy into electrical power, with advancements continuously improving their efficiency. However, their performance diminishes with distance from the Sun. In contrast, nuclear power systems, such as Radioisotope Thermoelectric Generators (RTGs) and nuclear fission reactors, offer higher energy density and consistent output regardless of solar conditions. This makes them particularly effective for deep space missions and long-duration operations. For missions that require high energy output over extended periods and beyond the reach of the Sun, nuclear power systems often provide a more efficient and reliable solution.
How Do Solar and Nuclear Power Systems Compare in Terms of Safety?
Safety is a critical factor when comparing solar and nuclear power systems for space missions. Solar power systems are generally considered safe, with the primary concerns related to the potential for damage to the panels from micrometeoroids or radiation. Solar panels have no radioactive materials and pose minimal risk to spacecraft or mission personnel. In contrast, nuclear power systems, while offering high energy density and reliability, come with more stringent safety considerations. Nuclear systems, such as RTGs, contain radioactive materials that require robust shielding to protect against radiation. Additionally, the potential for radioactive contamination in the event of a malfunction or accident must be carefully managed. Despite these risks, nuclear power systems are designed with rigorous safety protocols and containment measures to ensure that the benefits outweigh the potential hazards, particularly for long-duration and deep space missions.
What Are the Latest Advancements in Space Power Technologies?
Recent advancements in space power technologies are focused on increasing efficiency, durability, and versatility. In solar power, innovations include the development of high-efficiency photovoltaic cells, such as multi-junction cells that can capture a broader spectrum of sunlight and convert it into electrical power more effectively. Flexible and lightweight solar panels are also being developed to improve integration with spacecraft designs. In nuclear power, advancements include the Kilopower project, which is working on compact fission reactors capable of providing power for extended missions. Research into alternative nuclear technologies, such as fusion reactors, promises to offer even greater energy density and sustainability. Additionally, emerging technologies such as space-based solar power stations and wireless power transmission are being explored to address energy needs beyond traditional methods. These advancements aim to enhance the performance and reliability of power systems for future space missions.
How Do Spacecraft Power Systems Impact Mission Design?
Spacecraft power systems significantly impact mission design by influencing various aspects of spacecraft functionality, including weight, volume, and power distribution. The choice of power system affects the overall design, with solar panels requiring large surface areas for optimal energy capture, while nuclear power systems necessitate careful integration to manage radiation shielding and heat dissipation. Power systems also impact the spacecraft’s ability to support scientific instruments, communication equipment, and life support systems. For missions with high power demands or those venturing into deep space, the power system’s capacity and reliability become critical considerations. The design must ensure that the power system can meet the mission’s energy needs while maintaining structural integrity and operational efficiency. Effective power management and integration are essential to ensure the spacecraft’s success and longevity.
What Future Technologies Could Revolutionize Spacecraft Power?
Several future technologies hold the potential to revolutionize spacecraft power systems. One promising area is nuclear fusion power, which could provide an almost limitless and highly efficient energy source with minimal waste. Fusion reactors, if successfully developed, could offer significant advancements in power density and sustainability for long-duration missions. Another exciting technology is space-based solar power, where large arrays of solar panels in orbit could capture solar energy continuously and beam it to Earth or other spacecraft, overcoming the limitations of terrestrial solar power. Additionally, advancements in antimatter technology could lead to highly efficient propulsion systems with extremely high energy densities. Innovations in energy storage and wireless power transmission also have the potential to enhance power system flexibility and efficiency. These emerging technologies could transform the capabilities of spacecraft, enabling more ambitious missions and deeper exploration of space.