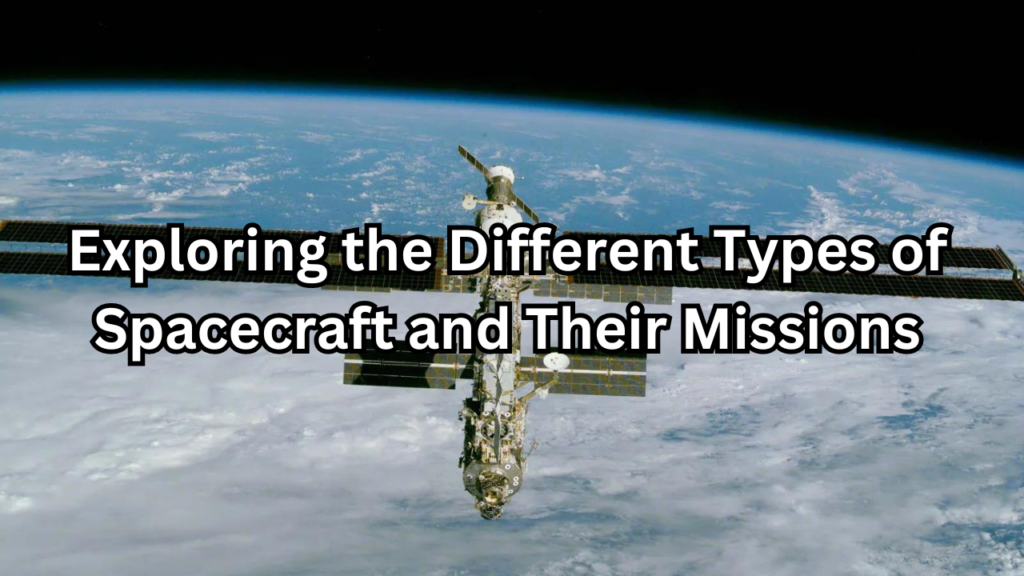
Table of Contents
Introduction
Overview of Spacecraft and Their Missions
Spacecraft and Their Missions, space exploration represents one of humanity’s most ambitious and awe-inspiring endeavors. From the moment humans first looked up at the stars with curiosity, we have dreamed of reaching beyond our terrestrial boundaries. This quest has led to the development of various spacecraft designed to explore, study, and understand the cosmos. Spacecraft have become our vehicles to unlock the mysteries of the universe, offering insights into the origins of our planet, the potential for life elsewhere, and the fundamental laws of physics. This journey has not only expanded our scientific knowledge but has also inspired generations to pursue careers in science, technology, engineering, and mathematics (STEM).
Importance of Understanding Different Spacecraft
Understanding the different types of spacecraft and their missions is crucial for several reasons. Firstly, it allows us to appreciate the complexity and ingenuity involved in space exploration. Each type of spacecraft is designed with specific goals in mind, from observing distant galaxies to conducting experiments on the International Space Station (ISS). By learning about these varied missions, we can better understand the challenges and innovations that drive space technology forward. Secondly, knowledge of spacecraft types helps in recognizing the contributions of space exploration to everyday life. Technologies developed for space missions often find applications in other fields, enhancing everything from medical devices to communication systems. Finally, as humanity stands on the brink of a new era of space exploration, including potential manned missions to Mars and beyond, understanding these technologies is essential for informed public discourse and support.
Structure of the Article
This article is structured to provide a comprehensive exploration of the different types of spacecraft and their missions. It is divided into the following sections:
- Historical Development of Spacecraft: This section will cover the early designs and significant technological advancements that have shaped modern spacecraft.
- Classification of Spacecraft by Mission Type: We will delve into the various categories of unmanned and manned spacecraft, highlighting key missions and their objectives.
- Classification by Propulsion System: This part will explain the different propulsion systems used in spacecraft, including chemical, electric, and nuclear propulsion.
- Classification by Orbit Type: Here, we will explore the different orbital paths taken by spacecraft, such as Low Earth Orbit (LEO), Medium Earth Orbit (MEO), and Geostationary Orbit (GEO).
- Specialized Spacecraft: This section will focus on spacecraft with unique functions, such as space telescopes, space stations, and future space habitats.
- Spacecraft Design and Construction: We will examine the materials, structural design, thermal control, and power systems crucial to spacecraft functionality.
- Communication and Navigation Systems: This part will discuss how spacecraft communicate with Earth and navigate through space, highlighting systems like the Deep Space Network (DSN).
- Challenges in Spacecraft Missions: We will address the various challenges faced during space missions, including radiation protection, microgravity effects, and mechanical failures.
- Future Trends in Spacecraft Design: The final section will look ahead to emerging trends and innovations in spacecraft design, such as reusable spacecraft, miniaturized satellites, and interstellar probes.
Historical Development of Spacecraft
Early Spacecraft Designs
The early designs of spacecraft were pioneering yet rudimentary, laying the foundation for the sophisticated technologies we see today. These initial concepts focused primarily on creating vehicles capable of reaching space and surviving the harsh conditions encountered beyond Earth’s atmosphere. Engineers and scientists worked under significant constraints, including limited technology, understanding of space conditions, and political pressures. The first spacecraft were typically small, simple in structure, and designed for short missions. They relied heavily on robust and redundant systems to ensure the success of their missions despite the limited computational power and materials available at the time. These early designs established the essential principles of spacecraft construction, such as modularity, reliability, and the ability to withstand extreme temperatures and radiation.
Sputnik and the Dawn of Space Exploration
The launch of Sputnik 1 by the Soviet Union on October 4, 1957, marked the dawn of space exploration and the beginning of the space race. Sputnik 1, the world’s first artificial satellite, was a metal sphere with four external radio antennas that transmitted radio pulses back to Earth. This historic event stunned the world, particularly the United States, and demonstrated the feasibility of sending objects into orbit around Earth. Sputnik’s successful deployment not only proved that humans could reach space but also set off a wave of technological advancements and competition between the superpowers. The satellite’s simple yet effective design included basic instrumentation for measuring temperature and pressure, providing invaluable data about the conditions in space. Sputnik 1’s launch was a pivotal moment that sparked widespread interest in space exploration and led to rapid developments in space technology.
Early NASA Missions
In response to the Soviet Union’s early achievements in space, the United States established the National Aeronautics and Space Administration (NASA) in 1958. NASA’s early missions focused on catching up with and surpassing Soviet space capabilities. The Mercury program was one of the first significant efforts, aiming to send a human into space and safely return them to Earth. The program achieved its goal with the successful flight of Alan Shepard in 1961, who became the first American in space. Following the Mercury program, NASA launched the Gemini program, which tested long-duration spaceflight and orbital rendezvous techniques critical for the upcoming Apollo missions. These early missions were marked by rapid learning, intense competition, and significant technological advancements, setting the stage for the historic Apollo moon landings.
Technological Advancements Over the Decades
Over the decades, spacecraft technology has seen remarkable advancements, driven by the continuous pursuit of exploring deeper into space and improving mission efficiency and safety. Innovations in materials science have led to the development of lighter and more durable spacecraft structures. Propulsion systems have evolved from traditional chemical rockets to include electric propulsion and ion thrusters, providing more efficient and longer-lasting power sources for deep space missions. Advances in computing and electronics have resulted in more sophisticated onboard systems capable of autonomous navigation, data processing, and real-time decision-making. Additionally, the miniaturization of technology has enabled the creation of small, yet powerful spacecraft such as CubeSats and nano-satellites, expanding the possibilities for scientific research and commercial applications. These technological advancements have not only made space exploration more feasible and cost-effective but have also paved the way for ambitious future missions, including human exploration of Mars and beyond.
Classification of Spacecraft by Mission Type
Unmanned Spacecraft
Unmanned spacecraft have been crucial in advancing our understanding of the universe without risking human life. These spacecraft include a variety of vehicles such as probes, satellites, and rovers, each designed for specific missions. Their tasks range from exploring distant planets and moons to observing Earth’s climate and transmitting communications. Unmanned missions can operate in environments too hostile for humans, collect data over long durations, and travel vast distances, making them invaluable tools in space exploration.
Probes
Probes are unmanned spacecraft designed to travel through space to collect data from distant celestial bodies and interstellar space. They are typically equipped with instruments to analyze the composition, atmosphere, and other physical characteristics of their targets. Probes can send back critical information to Earth, enabling scientists to make groundbreaking discoveries about our solar system and beyond.
Voyager Probes
The Voyager probes, Voyager 1 and Voyager 2, launched in 1977, are among the most famous space probes. These spacecraft were designed to take advantage of a rare planetary alignment that occurs once every 176 years, allowing them to visit Jupiter, Saturn, Uranus, and Neptune. The Voyager missions have provided humanity with its first close-up images of these planets and their moons. Voyager 1 has since entered interstellar space, providing valuable data on the outer limits of the sun’s influence.
Pioneer Probes
The Pioneer probes, including Pioneer 10 and Pioneer 11, were launched in the early 1970s to study the outer planets and the interstellar medium. Pioneer 10 was the first spacecraft to travel through the asteroid belt and make a close encounter with Jupiter, sending back images and data about the planet’s environment. Pioneer 11 followed a similar path, providing the first detailed images of Saturn and its rings. These missions paved the way for subsequent outer solar system exploration.
Satellites
Satellites are unmanned spacecraft that orbit Earth or other celestial bodies. They play a critical role in various applications, including communication, weather monitoring, navigation, and scientific research. Satellites can be geostationary, remaining over a fixed point on Earth, or they can follow low Earth orbits, providing global coverage. Their versatility and reliability make them essential for modern technology and science.
Communication Satellites
Communication satellites are designed to relay telecommunication signals, including television, radio, and internet data. They enable global communication by transmitting signals over vast distances, connecting people and businesses worldwide. These satellites are typically placed in geostationary orbit, ensuring consistent coverage over specific areas.
Weather Satellites
Weather satellites monitor Earth’s atmospheric conditions, providing critical data for weather forecasting and climate research. They can track storm development, measure temperature and humidity levels, and observe changes in the Earth’s surface. Weather satellites are vital for predicting natural disasters and understanding long-term climate trends.
Rovers
Rovers are unmanned, mobile spacecraft designed to explore the surface of other planets and moons. Equipped with scientific instruments, cameras, and robotic arms, rovers can conduct detailed analyses of their surroundings. They play a crucial role in studying the geology, climate, and potential habitability of extraterrestrial environments.
Mars Rovers: Curiosity and Perseverance
Curiosity and Perseverance are two of NASA’s most successful Mars rovers. Curiosity, launched in 2011, has been exploring Gale Crater, studying the planet’s climate and geology, and assessing whether Mars ever had the conditions to support microbial life. Perseverance, launched in 2020, aims to search for signs of past life and collect samples for potential return to Earth. Both rovers are equipped with advanced scientific instruments and have significantly advanced our understanding of Mars.
Lunar Rovers
Lunar rovers have been used in several missions to explore the Moon’s surface. The most famous of these are the Apollo Lunar Roving Vehicles (LRVs), used during the Apollo 15, 16, and 17 missions. These rovers allowed astronauts to travel further from their landing sites, conduct experiments, and collect samples. Modern lunar rover missions, such as China’s Yutu rovers, continue to explore the Moon, providing new insights into its composition and history.
Manned Spacecraft
Manned spacecraft are designed to carry astronauts into space, allowing humans to explore and conduct experiments beyond Earth. These spacecraft include vehicles for orbital missions, such as space stations, and those designed for deep space exploration. Manned missions have been pivotal in advancing human spaceflight capabilities and understanding the effects of space on the human body.
Orbital Spacecraft
Orbital spacecraft are designed to operate in Earth’s orbit, providing platforms for scientific research, technological development, and international collaboration. These include space stations, such as the International Space Station (ISS), where astronauts live and work for extended periods, conducting experiments that cannot be performed on Earth.
Apollo Missions
The Apollo missions were a series of manned spaceflights undertaken by NASA in the 1960s and 1970s, culminating in humans landing on the Moon. Apollo 11, in 1969, achieved the historic first moon landing, with astronauts Neil Armstrong and Buzz Aldrin becoming the first humans to walk on the lunar surface. The Apollo program demonstrated human capability to explore another celestial body and brought back valuable scientific data and samples from the Moon.
Space Shuttle Program
The Space Shuttle program, operational from 1981 to 2011, revolutionized human spaceflight by introducing reusable spacecraft. The Space Shuttle fleet conducted numerous missions, including deploying satellites, servicing the Hubble Space Telescope, and constructing the ISS. The program significantly advanced our understanding of living and working in space and laid the groundwork for future space exploration.
Deep Space Missions
Deep space missions aim to explore regions beyond Earth’s immediate vicinity, including the Moon, Mars, and other distant celestial bodies. These missions often involve long-duration flights and advanced spacecraft capable of operating in harsh environments. The goal is to expand human presence and scientific knowledge of the solar system and beyond.
Artemis Program
The Artemis program is NASA’s initiative to return humans to the Moon and establish a sustainable presence by the end of the decade. It aims to land the first woman and the next man on the lunar surface, using the knowledge and resources gained to prepare for future manned missions to Mars. Artemis will employ new technologies and international partnerships, advancing human exploration further into the solar system.
Future Mars Missions
Future Mars missions are focused on sending humans to explore the Red Planet. These missions aim to investigate the planet’s habitability, search for signs of past life, and establish the foundation for potential human settlement. They involve significant technological advancements in life support, propulsion, and habitat construction to ensure the safety and success of human explorers on Mars.
Classification by Propulsion System
Chemical Propulsion Spacecraft
Chemical propulsion remains one of the most widely used methods for spacecraft propulsion. This technology relies on the chemical reaction between propellants to generate thrust. The reaction produces high-speed exhaust gases that are expelled from the spacecraft, creating the force needed for movement. Chemical propulsion is known for its high thrust-to-weight ratio, making it suitable for launching spacecraft from Earth and for maneuvers that require significant acceleration. The two primary types of chemical propulsion systems are solid rocket engines and liquid rocket engines, each with distinct advantages and applications.
Traditional Rocket Engines
Traditional rocket engines utilize chemical reactions to produce thrust. These engines come in two main types: solid and liquid. Solid rocket engines use a solid propellant mixture that combusts to produce thrust, while liquid rocket engines burn liquid propellants, which are typically composed of a fuel and an oxidizer. Traditional rocket engines are valued for their simplicity and reliability. They have been fundamental in launching spacecraft and satellites, as well as in achieving orbit and interplanetary travel. Their proven track record in space missions underscores their continued importance in space exploration.
Solid vs. Liquid Propellants
Solid and liquid propellants each offer unique benefits and limitations. Solid propellants are pre-combined into a single substance and ignited to produce thrust. They are relatively simple and reliable, with fewer moving parts and lower maintenance requirements. However, they lack the ability to be throttled or shut off once ignited. Liquid propellants, on the other hand, are stored separately and mixed during combustion. They offer greater control over thrust, allowing for adjustments during flight, and can be shut down or restarted as needed. Despite their complexity and higher maintenance, liquid propellants are preferred for missions requiring precise control and multiple burns.
Electric Propulsion Spacecraft
Electric propulsion represents a more advanced technology that uses electrical energy to accelerate ions or other charged particles to generate thrust. Unlike chemical propulsion, which relies on chemical reactions, electric propulsion systems are characterized by their high efficiency and specific impulse, meaning they can produce thrust with less propellant. Electric propulsion is particularly suitable for deep-space missions where high efficiency is crucial. While it produces lower thrust compared to chemical propulsion, its efficiency allows spacecraft to achieve higher velocities over time.
Ion Thrusters
Ion thrusters are a type of electric propulsion system that accelerates ions using electric fields. These thrusters operate by ionizing a propellant, typically xenon gas, and using electromagnetic forces to expel the ions at high speeds. The result is a highly efficient propulsion system capable of producing thrust for extended periods. Ion thrusters are used in various space missions, including satellite station-keeping and deep-space exploration. Their high efficiency makes them ideal for missions that require long-duration propulsion with minimal fuel consumption.
Hall Effect Thrusters
Hall Effect thrusters are another form of electric propulsion that uses a magnetic field to accelerate ions. In these thrusters, a magnetic field generates an electric field that ionizes the propellant and accelerates the ions out of the engine. The interaction between the magnetic and electric fields creates a Hall Effect, which efficiently accelerates the ions to produce thrust. Hall Effect thrusters offer high efficiency and are commonly used for satellite propulsion and orbit transfers. They provide a balance between high specific impulse and manageable thrust levels, making them versatile for various space missions.
Nuclear Propulsion Spacecraft
Nuclear propulsion is an emerging technology that leverages nuclear reactions to produce thrust. This method includes two primary concepts: nuclear thermal propulsion (NTP) and nuclear electric propulsion (NEP). NTP systems use a nuclear reactor to heat a propellant, which is then expelled to create thrust. NEP systems generate electrical power from nuclear reactions to drive electric propulsion systems, such as ion thrusters. Nuclear propulsion promises significant advancements in spacecraft performance, offering higher specific impulses and potentially reducing travel time for deep-space missions. Research is ongoing to address challenges related to safety, efficiency, and radiation protection.
Concepts and Current Research
Current research in spacecraft propulsion includes developing advanced nuclear propulsion systems, improving electric propulsion technologies, and exploring hybrid approaches. Scientists and engineers are investigating new materials, designs, and fuels to enhance propulsion efficiency and capability. For instance, research into advanced ion thrusters and Hall Effect thrusters aims to increase their performance and reliability for future missions. Additionally, nuclear propulsion research focuses on developing reactors that are safe, efficient, and capable of operating in space environments. These advancements hold the potential to revolutionize space travel by enabling faster and more efficient missions to distant planets and beyond.
Potential Future Applications
The future applications of advanced propulsion technologies are vast and promising. Electric propulsion systems are likely to become standard for deep-space missions, enabling faster travel to Mars and other distant destinations. Nuclear propulsion could dramatically reduce travel times for interplanetary missions, making human exploration of the outer solar system more feasible. Additionally, advancements in propulsion technologies may lead to new mission types, such as crewed missions to the outer planets, asteroid mining, and space tourism. As research progresses, these technologies could transform our approach to space exploration, opening up new frontiers and opportunities for scientific discovery and commercial ventures.
Classification by Orbit Type
Low Earth Orbit (LEO) Spacecraft
Low Earth Orbit (LEO) spacecraft orbit at altitudes between approximately 160 to 2,000 kilometers above Earth’s surface. This proximity allows for frequent and rapid orbits, which is ideal for missions requiring close-up observations or continuous monitoring. LEO spacecraft are used for a variety of purposes, including Earth observation, communications, and scientific research. The relatively low altitude also facilitates easier access to space, reducing launch costs and enabling frequent resupply missions. Notable examples of LEO missions include the Hubble Space Telescope and various Earth observation satellites that provide valuable data for weather forecasting, environmental monitoring, and disaster response.
International Space Station (ISS)
The International Space Station (ISS) is a modular space station that orbits Earth at an altitude of approximately 400 kilometers. It serves as a microgravity laboratory where astronauts conduct scientific research across a range of disciplines, including biology, physics, astronomy, and materials science. The ISS is a collaborative project involving space agencies from the United States (NASA), Russia (Roscosmos), Europe (ESA), Japan (JAXA), and Canada (CSA). Its continuous presence in orbit since the year 2000 has made it a cornerstone of international cooperation in space exploration and a testbed for technologies and systems that will support future deep-space missions.
Earth Observation Satellites
Earth observation satellites are designed to monitor and collect data about Earth’s surface, atmosphere, and oceans. They play a crucial role in environmental management, weather forecasting, and disaster response. These satellites are equipped with various sensors and imaging systems to observe phenomena such as climate change, deforestation, urban development, and natural disasters. Earth observation satellites operate from various orbits, including LEO, to capture detailed and frequent images and data, which are then used by scientists, policymakers, and organizations to make informed decisions and address global challenges.
Medium Earth Orbit (MEO) Spacecraft
Medium Earth Orbit (MEO) spacecraft operate at altitudes ranging from about 2,000 to 20,000 kilometers above Earth’s surface. This orbit is typically used for navigation satellites and communication systems that require a balance between coverage area and signal latency. MEO provides a good compromise between the high resolution of LEO and the extensive coverage of Geostationary Orbit (GEO). Notable examples of MEO missions include global navigation systems such as GPS (Global Positioning System), Galileo, and GLONASS, which provide accurate positioning and timing information for various applications, including navigation, surveying, and military operations.
GPS Satellites
GPS satellites are part of the Global Positioning System, a constellation of satellites that provide precise location and timing information to users around the world. These satellites orbit Earth in Medium Earth Orbit (MEO) and transmit signals that can be received by GPS devices on the ground. The system enables applications such as navigation, mapping, and tracking for both civilian and military purposes. GPS satellites work in conjunction with ground-based systems to calculate the position of receivers with high accuracy, making them an essential tool for modern navigation and location-based services.
Geostationary Orbit (GEO) Spacecraft
Geostationary Orbit (GEO) spacecraft orbit at an altitude of approximately 35,786 kilometers above Earth’s equator. At this altitude, the spacecraft’s orbital period matches Earth’s rotation period, allowing them to remain fixed over a specific point on the Earth’s surface. This stationary position is ideal for communication satellites, weather monitoring, and broadcasting services. GEO satellites provide continuous coverage of specific regions, making them crucial for telecommunications, satellite television, and real-time weather observations.
Communication Satellites
Communication satellites are designed to relay telecommunication signals, such as television, radio, internet, and phone services, across long distances. These satellites can be positioned in various orbits, including GEO, MEO, and LEO, depending on their intended purpose. Communication satellites facilitate global connectivity by transmitting signals between ground stations and other satellites, enabling real-time communication and data transfer. They play a vital role in modern communication infrastructure, supporting everything from emergency response to everyday media consumption.
Highly Elliptical Orbit (HEO) Spacecraft
Highly Elliptical Orbit (HEO) spacecraft follow elliptical paths that bring them close to Earth at perigee and far away at apogee. This type of orbit is used to provide extended coverage over specific regions, particularly at high latitudes. HEO spacecraft are valuable for applications that require prolonged observation of areas with limited visibility from other orbits, such as polar regions. Examples include Molniya satellites, which are designed for communication and weather monitoring in high-latitude areas, where other orbital paths might be less effective.
Molniya Satellites
Molniya satellites are a specific type of spacecraft placed into a Highly Elliptical Orbit (HEO) known as a Molniya orbit. This orbit has a high inclination and is designed to provide extensive coverage of high-latitude regions, such as Russia and northern Canada. Molniya satellites are used for communication, weather monitoring, and reconnaissance in areas where geostationary satellites would not provide adequate coverage. The orbit allows these satellites to spend a significant portion of their orbit over the desired high-latitude areas, making them essential for services and data collection in these regions.
Specialized Spacecraft
Space Telescopes
Space telescopes are observational instruments positioned in space to bypass Earth’s atmospheric distortion and capture clearer images of celestial objects. By operating outside of the Earth’s atmosphere, these telescopes avoid the interference caused by atmospheric turbulence, weather conditions, and light pollution. Space telescopes can observe a wide range of electromagnetic wavelengths, from visible light to infrared and ultraviolet, providing valuable data about the universe’s structure, formation, and evolution. Their vantage point allows for more detailed and accurate observations of distant galaxies, stars, and other cosmic phenomena, significantly enhancing our understanding of the universe.
Hubble Space Telescope
Launched in 1990, the Hubble Space Telescope has been one of the most influential tools in modern astronomy. Positioned in Low Earth Orbit (LEO), Hubble has provided breathtaking images and critical data about distant galaxies, nebulae, and exoplanets. Its observations have led to significant discoveries, including the acceleration of the universe’s expansion and the detailed analysis of cosmic structures. Hubble’s advanced instruments allow it to capture high-resolution images across various wavelengths, contributing to a wide range of scientific fields, from the study of star formation to the examination of the cosmic microwave background.
James Webb Space Telescope
The James Webb Space Telescope (JWST), launched in December 2021, is designed to be the successor to the Hubble Space Telescope. Positioned at the second Lagrange point (L2), approximately 1.5 million kilometers from Earth, JWST is equipped with advanced instruments to observe the universe in infrared wavelengths. This capability allows JWST to peer through dust clouds and study the formation of the earliest galaxies, stars, and planetary systems. Its larger primary mirror and advanced technology promise to provide unprecedented detail in observations, offering new insights into the formation of the universe and the potential for life in distant exoplanetary systems.
Space Stations
Space stations are habitable artificial satellites that orbit Earth or other celestial bodies, providing a platform for scientific research, technology testing, and international cooperation. Unlike spacecraft, which are designed for short-term missions, space stations are intended for long-term habitation and operations. They serve as laboratories for experiments in microgravity, which cannot be conducted on Earth, and play a crucial role in studying the effects of prolonged spaceflight on the human body. Space stations also support ongoing research in space technology, materials science, and biology, contributing to our understanding of living and working in space.
International Space Station
The International Space Station (ISS) is a multi-national collaborative project that orbits Earth at an altitude of approximately 400 kilometers. Since its first module was launched in 1998, the ISS has become a cornerstone of international space exploration, involving contributions from NASA, Roscosmos, ESA, JAXA, and CSA. The ISS serves as a microgravity laboratory where astronauts conduct experiments across various scientific disciplines, including biology, physics, and astronomy. It also functions as a testbed for technologies and systems that will be essential for future deep-space missions. The ISS represents one of the most ambitious international collaborations in space exploration, demonstrating the benefits of global cooperation in advancing human spaceflight.
Tiangong Space Station
Tiangong, meaning “Heavenly Palace,” is China’s modular space station currently under construction in Low Earth Orbit. Initiated by the China National Space Administration (CNSA), Tiangong aims to provide a platform for scientific research and international cooperation. The space station consists of a core module and multiple laboratory modules, designed to support a variety of scientific experiments and technology demonstrations. With its emphasis on autonomous operation and advanced technologies, Tiangong represents China’s significant step forward in space exploration and its commitment to establishing a long-term human presence in space.
Space Habitats
Space habitats are specially designed living environments intended for humans to live and work in space for extended periods. These habitats are equipped with life support systems to provide air, water, and food, and they include facilities for conducting research and maintaining human health. Space habitats can be part of larger space stations or stand-alone modules, and they are critical for future long-duration missions to the Moon, Mars, and beyond. Designing effective space habitats involves addressing challenges such as radiation protection, psychological well-being, and sustainable resource management to ensure the health and productivity of astronauts during their missions.
Concepts for Future Mars and Moon Bases
Future Mars and Moon bases are envisioned as key components of humanity’s expansion into the solar system. These bases will serve as outposts for scientific research, resource utilization, and potential human settlement. Concepts for Moon bases often include constructing habitats using lunar regolith, establishing systems for extracting water and oxygen, and developing technologies for living and working on the Moon’s surface. Mars base concepts focus on creating self-sustaining habitats with advanced life support systems, radiation shielding, and in-situ resource utilization to support long-term missions. Both types of bases aim to build on current technology and research to enable sustainable human presence and exploration beyond Earth.
Spacecraft Design and Construction
Materials and Structural Design
Materials and structural design are critical components in spacecraft engineering, ensuring that spacecraft can withstand the harsh conditions of space travel. Spacecraft must be constructed from materials that are both lightweight and durable, capable of enduring extreme temperatures, radiation, and mechanical stresses. Common materials include advanced composites, aluminum alloys, and titanium, chosen for their strength-to-weight ratio and resistance to space environments. The structural design must accommodate thermal expansion and contraction, vibrational forces during launch, and the vacuum of space. Effective design strategies involve the use of modular components and advanced analysis techniques to ensure structural integrity and mission success.
Thermal Control Systems
Thermal control systems are essential for managing the temperature of spacecraft and their components, maintaining operational conditions despite the extreme temperatures of space. These systems use a combination of passive and active methods to regulate temperature. Passive thermal control involves materials and coatings that reflect or absorb heat, such as thermal blankets and radiators. Active thermal control systems include heaters and fluid loops that circulate coolant to dissipate excess heat. Together, these systems ensure that spacecraft components operate within their specified temperature ranges, preventing overheating or freezing and ensuring the proper functioning of scientific instruments and onboard systems.
Power Systems
Power systems are crucial for providing and managing the energy required for spacecraft operations. Spacecraft typically rely on two main power sources: solar panels and radioisotope thermoelectric generators (RTGs). Solar panels convert sunlight into electrical energy, which is used to power spacecraft systems and charge batteries for use during periods of eclipse. RTGs provide a steady power supply using the heat generated from the decay of radioactive isotopes. Power systems must be designed to ensure reliable energy generation, distribution, and storage, as well as to accommodate the varying power needs of different spacecraft components and instruments.
Solar Panels
Solar panels are a primary source of power for many spacecraft, converting sunlight into electrical energy through photovoltaic cells. These panels are often mounted on the spacecraft’s exterior and oriented to maximize exposure to the Sun. The electricity generated by the solar panels is used to power spacecraft systems and charge onboard batteries, which supply energy during periods when the spacecraft is in the Earth’s shadow or away from the Sun. Solar panels must be designed to withstand the harsh space environment, including radiation and micrometeoroid impacts, and must be deployable and stowable to fit within the spacecraft’s launch configuration.
Radioisotope Thermoelectric Generators (RTGs)
Radioisotope Thermoelectric Generators (RTGs) provide a reliable and long-lasting power source for spacecraft operating far from the Sun, where solar panels are less effective. RTGs use the heat produced by the natural radioactive decay of isotopes, such as plutonium-238, to generate electricity through thermoelectric materials. This heat is converted into electrical power using thermocouples, which maintain a continuous and stable power output over long durations. RTGs are used in deep-space missions, such as those to the outer planets or interstellar space, where solar energy is insufficient. Their long operational life and reliability make them ideal for powering spacecraft and instruments in challenging and distant environments.
Communication and Navigation Systems
Telemetry and Tracking
Telemetry and tracking are essential for monitoring and controlling spacecraft during their missions. Telemetry refers to the collection and transmission of data from the spacecraft to Earth, including information on spacecraft health, system status, and scientific observations. This data is transmitted through communication systems and analyzed by ground control teams to ensure the spacecraft operates as expected. Tracking involves determining the spacecraft’s position and trajectory using ground-based or space-based tracking systems. Accurate tracking allows mission controllers to predict the spacecraft’s path, manage its operations, and make necessary adjustments to its course or velocity. Together, telemetry and tracking ensure the spacecraft remains on its intended path and can carry out its mission objectives.
Deep Space Network (DSN)
The Deep Space Network (DSN) is a global network of large antennas and communication facilities operated by NASA to support space missions beyond Earth’s orbit. The DSN consists of three primary complexes located in California, Spain, and Australia, strategically positioned around the globe to maintain continuous communication with spacecraft as Earth rotates. The network provides vital support for deep-space missions, including tracking spacecraft, receiving scientific data, and sending commands. The DSN’s large, high-precision antennas are capable of communicating with spacecraft across vast distances, enabling deep-space exploration and the collection of valuable data from missions to the Moon, Mars, and beyond.
Autonomous Navigation Systems
Autonomous navigation systems are designed to enable spacecraft to operate independently of constant ground control input by using onboard sensors and algorithms to determine their position, orientation, and trajectory. These systems use data from various sensors, such as star trackers, gyroscopes, and radar, to calculate the spacecraft’s position and adjust its course as needed. Autonomous navigation is crucial for missions where real-time communication with Earth is limited due to distance or time delays, such as deep-space missions. By allowing spacecraft to make navigation decisions and execute maneuvers autonomously, these systems enhance mission efficiency, reduce the need for constant ground intervention, and enable complex operations such as landing and docking with minimal human oversight.
Challenges in Spacecraft Missions
Radiation Protection
Radiation protection is a critical aspect of spacecraft design to safeguard both electronic systems and human occupants from the harmful effects of cosmic and solar radiation. Spacecraft are exposed to high levels of radiation in space, which can damage electronic components, degrade materials, and pose health risks to astronauts. To mitigate these effects, spacecraft are equipped with shielding materials, such as polyethylene and aluminum, that absorb or deflect radiation. Additionally, mission planning includes strategies to minimize radiation exposure, such as scheduling spacewalks during periods of lower solar activity and using protective clothing. Radiation protection measures are essential for ensuring the long-term reliability of spacecraft and the safety of crew members on deep-space missions.
Microgravity Effects
Microgravity, the condition of experiencing very low gravitational forces, is a fundamental aspect of space missions and has significant effects on both biological organisms and physical processes. In microgravity environments, fluid behavior, material properties, and biological functions can differ drastically from those observed on Earth. For example, fluids tend to form spherical shapes, and combustion processes behave differently. This unique environment allows scientists to conduct experiments that are not possible on Earth, leading to advancements in materials science, medicine, and biology. However, microgravity also presents challenges, such as muscle atrophy and bone density loss in astronauts. Addressing these effects involves designing countermeasures, such as exercise regimens and specialized equipment, to maintain astronaut health and performance during long-duration missions.
Mechanical Failures and Redundancy
Mechanical failures in spacecraft can have serious consequences, potentially jeopardizing mission success and crew safety. To address this risk, spacecraft are designed with redundancy in critical systems and components. Redundancy involves incorporating duplicate systems or backup components that can take over if the primary systems fail. For example, spacecraft may have multiple power sources, communication systems, and life support mechanisms to ensure continuous operation. Regular testing and maintenance are also part of the strategy to minimize the likelihood of failures. By building redundancy into the design and operation of spacecraft, mission planners enhance reliability, reduce the impact of unexpected failures, and increase the overall success rate of space missions.
Future Trends in Spacecraft Design
Reusable Spacecraft
Reusable spacecraft represent a significant advancement in space exploration, aiming to reduce the cost of access to space by enabling multiple missions with the same vehicle. Unlike traditional expendable spacecraft, reusable designs are engineered to be recovered, refurbished, and launched again, which can lead to substantial savings in both time and resources. Key features of reusable spacecraft include robust landing systems, modular components, and efficient refurbishment processes. By reusing spacecraft, space agencies and private companies can increase the frequency of space missions, lower costs, and accelerate the pace of exploration and commercial activities in space.
SpaceX’s Starship
SpaceX’s Starship is a fully reusable spacecraft and rocket system designed for a wide range of missions, including crewed spaceflights to the Moon and Mars. The Starship system consists of two stages: the Super Heavy booster, which provides initial thrust during launch, and the Starship spacecraft, which carries payloads and passengers. Starship aims to reduce the cost of space travel by allowing for rapid turnaround between flights. Its design includes advanced materials, aerodynamic features, and a powerful engine system to support deep-space missions. SpaceX envisions Starship as a key element in their plans for interplanetary colonization and space tourism.
Blue Origin’s New Shepard
Blue Origin’s New Shepard is a suborbital spacecraft designed to provide brief, weightless spaceflights for tourists and scientific experiments. The spacecraft consists of a rocket booster and a crew capsule, which are designed to be reused multiple times. New Shepard is named after Alan Shepard, the first American in space. The vehicle’s mission profile includes a vertical launch and landing, which allows for precision recovery and reuse. New Shepard aims to make space more accessible to the general public by offering commercial space tourism experiences, as well as providing a platform for scientific research in microgravity.
Miniaturized Spacecraft
Miniaturized spacecraft are compact, cost-effective space vehicles designed for a variety of missions, including Earth observation, scientific research, and technology demonstration. These spacecraft, which include CubeSats and NanoSats, leverage advancements in miniaturization and integration to perform tasks traditionally reserved for larger, more expensive satellites. Their small size and lower cost enable high-frequency, low-risk missions and provide opportunities for educational institutions and small businesses to participate in space exploration. Despite their size, miniaturized spacecraft can carry sophisticated sensors and instruments, making them valuable tools for scientific discovery and technology testing.
CubeSats and NanoSats
CubeSats and NanoSats are types of miniaturized spacecraft designed to be deployed in low Earth orbit and beyond. CubeSats are small, modular satellites with standardized dimensions, typically measuring 10x10x10 centimeters per unit (1U) and can be configured in multiple-unit sizes (e.g., 3U, 6U). NanoSats are even smaller, with a mass of less than 10 kilograms. Both types of satellites are used for a range of applications, including Earth observation, communications, and scientific research. Their compact size and affordability make them accessible for academic institutions, startups, and international partners, expanding opportunities for space exploration and technology development.
Interstellar Spacecraft
Interstellar spacecraft are designed to travel beyond the solar system, aiming to explore other star systems and potentially habitable exoplanets. The development of interstellar spacecraft poses significant technical challenges, including propulsion systems capable of achieving a substantial fraction of the speed of light, long-duration life support systems, and advanced communication technologies. Concepts for interstellar travel include propulsion methods such as nuclear fusion, antimatter engines, and light sails. The goal of interstellar missions is to expand our understanding of the universe and search for extraterrestrial life, potentially paving the way for humanity’s future beyond the solar system.
Breakthrough Starshot Initiative
The Breakthrough Starshot Initiative is an ambitious project aimed at developing a fleet of small, lightweight spacecraft capable of traveling to the nearest star system, Alpha Centauri, within a human lifetime. The initiative proposes using powerful ground-based lasers to propel tiny, light sail-equipped spacecraft to speeds approaching 20% of the speed of light. The goal is to reach Alpha Centauri in just over 20 years, gathering data on potential habitable planets and interstellar environments. Breakthrough Starshot represents a bold effort to advance interstellar travel technology and explore the feasibility of reaching distant star systems with current scientific and engineering capabilities.
Conclusion
In conclusion, the evolution of spacecraft and their missions has profoundly expanded our understanding of the universe and our ability to explore it. From the early days of space exploration, characterized by pioneering missions and basic spacecraft designs, to the sophisticated technologies of today, including reusable spacecraft and interstellar probes, each advancement has brought us closer to uncovering the mysteries of space. By continually pushing the boundaries of engineering and technology, we are not only enhancing our capabilities for exploration but also paving the way for future discoveries that will shape humanity’s place in the cosmos.
Summary of Different Types of Spacecraft and Their Missions
Throughout the history of space exploration, various types of spacecraft have been developed to fulfill specific mission objectives. Unmanned spacecraft, such as probes and satellites, have been instrumental in gathering data from distant planets and observing Earth. Manned spacecraft, including orbital vehicles and deep space missions, have enabled human presence and scientific research in space. Advances in propulsion technologies, such as chemical, electric, and nuclear systems, have expanded the range and capabilities of these spacecraft. Spacecraft are designed for diverse environments, from low Earth orbit to deep space, and feature systems like thermal control, power management, and radiation protection to ensure mission success. As technology continues to advance, new concepts and innovations will further enhance our ability to explore and understand the universe.
The Future of Space Exploration
The future of space exploration holds exciting possibilities as new technologies and mission concepts are developed. Reusable spacecraft, such as SpaceX’s Starship and Blue Origin’s New Shepard, are expected to revolutionize access to space, making it more cost-effective and frequent. Miniaturized spacecraft, like CubeSats, will continue to expand opportunities for scientific research and technology demonstration. Future missions will likely focus on deep-space exploration, including interstellar travel, with initiatives like Breakthrough Starshot aiming to reach other star systems. The continued advancement of propulsion technologies, radiation protection, and autonomous systems will be crucial for enabling long-duration missions and human settlement on other planets. As we look forward, the integration of international collaboration, private sector innovation, and cutting-edge research will drive the next era of exploration and discovery in space.
Frequently Asked Questions (FAQs)
What are the main types of spacecraft?
Spacecraft can be broadly categorized into several types based on their design and mission objectives. The main types include unmanned spacecraft, such as probes and satellites, which are used for data collection and Earth observation. Manned spacecraft are designed to carry astronauts and support human missions, including orbital vehicles like the International Space Station (ISS) and deep space missions such as the Apollo program. Additionally, there are specialized spacecraft, including miniaturized satellites like CubeSats, reusable spacecraft like SpaceX’s Starship, and interstellar probes aimed at exploring beyond our solar system.
How do spacecraft navigate in space?
Spacecraft navigate in space using a combination of onboard sensors and ground-based tracking systems. Autonomous navigation systems onboard spacecraft use data from star trackers, gyroscopes, and radar to determine their position and trajectory. Ground control stations track the spacecraft’s position using radio signals and tracking data from networks like the Deep Space Network (DSN). Navigation involves calculating precise trajectories, making course adjustments, and managing orbital maneuvers to ensure the spacecraft remains on its intended path and successfully completes its mission objectives.
What are the challenges faced by spacecraft in deep space missions?
Deep space missions present several significant challenges. One major challenge is the harsh and unpredictable environment of space, which includes extreme temperatures, high radiation levels, and the vacuum of space. Spacecraft must be designed with robust radiation shielding, thermal control systems, and durable materials to withstand these conditions. Additionally, the vast distances involved in deep space travel create communication delays and difficulties in real-time control, requiring spacecraft to have autonomous systems for navigation and operation. Long-duration missions also pose challenges for maintaining spacecraft systems and ensuring the reliability of scientific instruments and life support systems.
How has spacecraft technology evolved over the years?
Spacecraft technology has evolved significantly since the early days of space exploration. Initial spacecraft were relatively simple, with limited capabilities and short mission durations. Over time, advancements in materials science, propulsion systems, and electronics have led to the development of more sophisticated spacecraft. Key milestones include the introduction of reusable spacecraft, such as the Space Shuttle, and the development of miniaturized satellites, like CubeSats. Modern spacecraft benefit from advanced technologies such as autonomous navigation systems, high-efficiency propulsion methods, and improved communication systems. These advancements have expanded mission possibilities, enhanced scientific research, and reduced the costs of space exploration.
What are the most significant future trends in spacecraft design?
Significant future trends in spacecraft design include the development of reusable spacecraft, which aim to lower the cost of space access and increase mission frequency. Innovations in propulsion technologies, such as advanced ion thrusters and potential nuclear propulsion, are expected to enable faster and more efficient space travel. The integration of autonomous systems and artificial intelligence will enhance spacecraft capabilities for deep space exploration and long-duration missions. Additionally, there is a growing focus on miniaturization, with CubeSats and other small satellites playing a larger role in scientific research and technology testing. The future of spacecraft design also includes concepts for interstellar travel and the establishment of human settlements on other planets, driving the next frontier in space exploration.