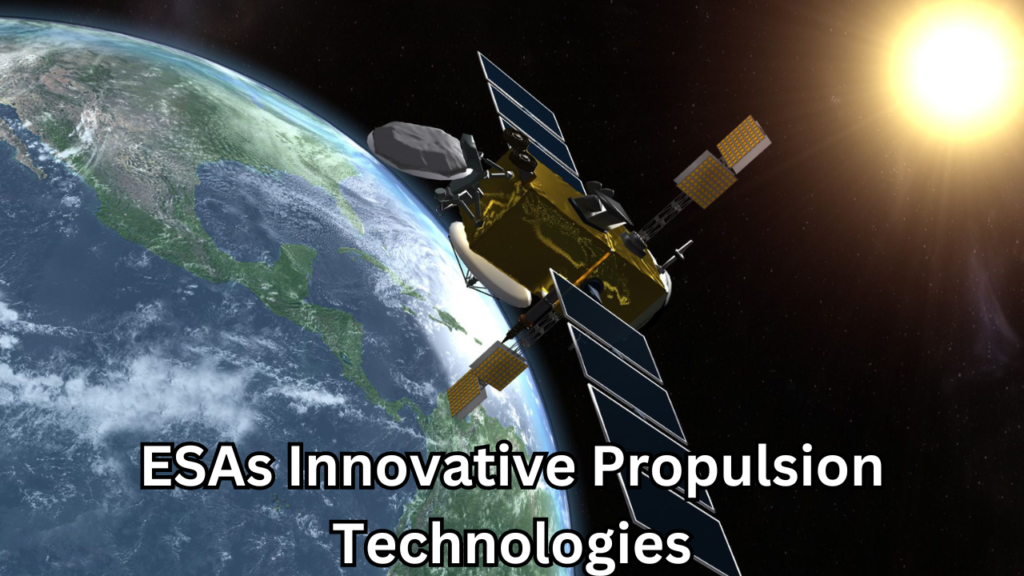
Table of Contents
Introduction
Overview of ESA and its Role in Space Exploration
The ESAs Innovative Propulsion Technologies is a pivotal organization in the realm of space exploration, representing the collective efforts of 22 European countries. Established in 1975, ESA’s mission is to shape the development of Europe’s space capability and ensure that investment in space continues to deliver benefits to the citizens of Europe and the world. ESA’s diverse portfolio encompasses a wide range of activities, including scientific exploration, Earth observation, telecommunications, navigation, and human spaceflight. By fostering international cooperation, advancing scientific knowledge, and developing cutting-edge technologies, ESA plays a crucial role in both advancing humanity’s understanding of space and contributing to practical applications that benefit life on Earth.
Importance of ESAs Innovative Propulsion Technologies
Propulsion technologies are the backbone of space exploration and satellite deployment. These technologies provide the necessary thrust to launch spacecraft from Earth, maneuver them in space, and control their trajectories over extended missions. The effectiveness, efficiency, and innovation in propulsion systems directly impact the feasibility and success of space missions. Whether it’s placing satellites into orbit, sending probes to distant planets, or ensuring the safe travel of astronauts, propulsion systems are integral to overcoming the immense distances and gravitational challenges of space. Advances in propulsion technology not only enable more ambitious missions but also enhance the sustainability and cost-effectiveness of space operations.
Purpose of the Article
This article aims to delve into the innovative propulsion technologies developed and utilized by the European Space Agency (ESA). By exploring various propulsion methods, their applications, and the technological advancements achieved by ESA, this article seeks to provide a comprehensive understanding of how these technologies are transforming space exploration. From traditional chemical propulsion to cutting-edge electric and green propulsion systems, the discussion will cover the full spectrum of ESA’s propulsion innovations. The article will also highlight ESA’s contributions to international space missions, the environmental implications of propulsion technologies, and future trends that could revolutionize space travel. Ultimately, this article is intended to inform and inspire those interested in the dynamic field of space propulsion, showcasing ESA’s pivotal role in pushing the boundaries of what is possible in space exploration.
Historical Context
Early Propulsion Methods in Space Exploration
The dawn of space exploration in the mid-20th century was characterized by the use of rudimentary propulsion methods, primarily chemical rockets. These rockets relied on the combustion of propellants to produce high-speed exhaust gases, generating the thrust needed to overcome Earth’s gravitational pull. The V-2 rocket, developed during World War II by Germany, is a notable example of early rocket technology that laid the groundwork for future space missions. After the war, this technology was further developed and refined, leading to the launch of Sputnik 1 by the Soviet Union in 1957, the first artificial satellite to orbit the Earth.
Chemical propulsion systems, such as those used in the Redstone and Atlas rockets, played a critical role in the early space missions of the United States, including the Mercury and Gemini programs. These systems utilized liquid propellants, typically a combination of a fuel (like kerosene) and an oxidizer (such as liquid oxygen), to achieve the necessary thrust. The successful use of these early propulsion methods enabled the historic Apollo missions, culminating in the first manned moon landing in 1969.
Evolution of Propulsion Technology at ESA
The European Space Agency (ESA) has been at the forefront of developing and advancing propulsion technologies since its inception in 1975. Initially, ESA relied on conventional chemical propulsion systems similar to those used by other space agencies. The Ariane 1, ESA’s first launch vehicle, employed liquid-fueled engines that demonstrated the reliability and effectiveness of chemical propulsion. As ESA’s ambitions grew, so did its propulsion capabilities, leading to the development of the Ariane 4 and Ariane 5 rockets, which became known for their versatility and high payload capacities.
Recognizing the limitations of traditional chemical propulsion, ESA began investing in alternative technologies. This led to significant advancements in electric propulsion, which uses electrical energy to accelerate ions, providing a much higher efficiency compared to chemical systems. ESA’s SMART-1 mission, launched in 2003, was a milestone in this regard, utilizing an ion thruster to reach the moon. This mission demonstrated the viability of electric propulsion for deep space exploration and paved the way for its use in other missions, such as the BepiColombo mission to Mercury.
In addition to electric propulsion, ESA has been exploring green propulsion technologies, which aim to reduce the environmental impact of space missions. These technologies use non-toxic propellants, enhancing safety and sustainability. ESA’s commitment to innovation is also evident in its research on hybrid propulsion systems and solar sails, which harness solar energy for propulsion, offering a promising alternative for long-duration missions.
Today, ESA continues to push the boundaries of propulsion technology, focusing on developing advanced propulsion systems that will enable more ambitious missions, reduce costs, and ensure the sustainability of space exploration. The evolution of propulsion technology at ESA reflects its ongoing commitment to innovation, international collaboration, and scientific excellence in the quest to explore the cosmos.
Chemical Propulsion
Traditional Chemical Propulsion
Basics of Chemical Propulsion
Chemical propulsion is the most traditional and widely used method of powering rockets and spacecraft. It operates on the principle of Newton’s third law of motion: for every action, there is an equal and opposite reaction. In a chemical propulsion system, a chemical reaction occurs between a fuel and an oxidizer, producing hot gases. These gases are expelled through a nozzle at high speed, generating thrust that propels the spacecraft forward.
Chemical propulsion systems are generally classified into two types: liquid propulsion and solid propulsion. In liquid propulsion, the fuel and oxidizer are stored separately and pumped into a combustion chamber where they mix and ignite. This type allows for better control over the engine’s thrust and can be throttled, shut down, and restarted. In contrast, solid propulsion involves a single solid propellant that contains both fuel and oxidizer. Solid rocket engines are simpler and more reliable but offer less control over thrust and cannot be stopped once ignited.
Examples of Traditional Chemical Propulsion Used by ESA
ESA has a long history of utilizing traditional chemical propulsion in its launch vehicles. One of the earliest examples is the Ariane 1 rocket, which used liquid propellant engines for its first and second stages, combining nitrogen tetroxide as the oxidizer and unsymmetrical dimethylhydrazine (UDMH) as the fuel. The Ariane 1 successfully launched numerous payloads into orbit, establishing ESA’s capability in space launch operations.
The Ariane 4, introduced in 1988, became one of ESA’s most successful launch vehicles, thanks to its versatile design that could accommodate various payload configurations. It utilized a combination of liquid and solid propellants, with the core stages powered by liquid propellants and additional solid rocket boosters for extra thrust. This hybrid approach enhanced the rocket’s performance and reliability, making it a workhorse for ESA’s launch operations until its retirement in 2003.
The Ariane 5, ESA’s current heavy-lift launch vehicle, continues the tradition of using liquid propulsion systems. Its core stage employs a Vulcain engine, which burns liquid oxygen and liquid hydrogen, while the two solid rocket boosters provide the additional thrust needed during liftoff. The Ariane 5 has been instrumental in deploying satellites, space probes, and cargo for the International Space Station (ISS).
Advances in Chemical Propulsion
Recent Innovations in Chemical Propulsion at ESA
While traditional chemical propulsion systems have been effective, ESA has continuously sought to improve their efficiency, performance, and environmental impact. One notable innovation is the development of the Vinci engine, designed for the upper stages of the next-generation Ariane 6 launch vehicle. The Vinci engine uses an expander cycle, where the liquid hydrogen used as fuel also cools the engine’s combustion chamber and nozzle before being burned. This design enhances engine efficiency and allows for multiple restarts, enabling more flexible mission profiles and precise satellite deployments.
ESA is also exploring the use of green propellants to replace toxic substances like hydrazine, which poses significant environmental and safety risks. The LISA Pathfinder mission, for instance, tested a propulsion system using a green propellant based on ammonium dinitramide (ADN), which is less hazardous and offers similar performance to traditional hydrazine-based systems.
Advantages and Challenges
The primary advantage of chemical propulsion lies in its high thrust, which is essential for overcoming Earth’s gravity during launch. Liquid propulsion systems, in particular, offer significant flexibility, allowing for thrust modulation and engine restarts. Solid propulsion systems, on the other hand, are valued for their simplicity and reliability.
However, chemical propulsion also presents several challenges. The efficiency of chemical rockets, measured as specific impulse (ISP), is relatively low compared to other propulsion methods, such as electric propulsion. This means that more propellant is needed for a given mission, increasing the launch mass and cost. Additionally, the use of toxic propellants like hydrazine raises environmental and safety concerns, prompting the need for safer alternatives.
Despite these challenges, ongoing advancements in chemical propulsion technology at ESA continue to enhance the performance and sustainability of their launch vehicles. By integrating innovations like the Vinci engine and green propellants, ESA aims to address the limitations of traditional chemical propulsion while maintaining the high thrust capabilities required for successful space missions.
Electric Propulsion
Introduction to Electric Propulsion
Definition and Principles
Electric propulsion is a method of propelling spacecraft that utilizes electrical energy to accelerate propellant ions to produce thrust. Unlike traditional chemical propulsion, which relies on chemical reactions to generate high-speed exhaust gases, electric propulsion uses electric or magnetic fields to accelerate ions to very high velocities. This results in a much more efficient use of propellant, enabling spacecraft to carry less fuel and more scientific instruments or other payloads.
The basic principle of electric propulsion involves ionizing a propellant gas, such as xenon, and then accelerating these ions through an electric or magnetic field to produce thrust. The ions are expelled at high velocities, generating a continuous, albeit low, thrust. While the thrust produced by electric propulsion is much lower than that of chemical propulsion, it can be sustained over long periods, allowing spacecraft to reach high speeds over time.
Why Electric Propulsion is a Game-Changer
Electric propulsion is considered a game-changer in space exploration for several reasons. First, its high efficiency, measured in specific impulse (ISP), is significantly greater than that of chemical propulsion. This means that spacecraft equipped with electric propulsion systems can achieve much higher velocities with the same amount of propellant, extending mission durations and enabling more ambitious missions.
Second, the reduced propellant requirement translates to lower launch mass and cost, allowing for more efficient use of launch vehicles and potentially reducing the overall cost of space missions. This is particularly important for deep space missions, where carrying large amounts of chemical propellant would be impractical.
Finally, electric propulsion enables precise control of spacecraft trajectories and orbits, facilitating complex maneuvers and station-keeping operations. This makes it ideal for applications such as satellite station-keeping, interplanetary missions, and formation flying.
Types of Electric Propulsion
Ion Thrusters
Ion thrusters are a type of electric propulsion system that uses electrostatic fields to accelerate ions to produce thrust. In an ion thruster, a propellant gas, typically xenon, is ionized by bombarding it with electrons. The resulting positive ions are then accelerated through a series of grids with high electric potential differences, creating a stream of ions expelled at high velocity.
Ion thrusters are known for their high efficiency and specific impulse, making them suitable for long-duration missions. However, they produce relatively low thrust, requiring extended operation to achieve significant velocity changes.
Hall Effect Thrusters
Hall effect thrusters, another type of electric propulsion, use a combination of electric and magnetic fields to accelerate ions. In a Hall thruster, electrons are trapped in a circular path by a radial magnetic field, creating a Hall current. This current ionizes the propellant gas, and the ions are then accelerated by an electric field between an anode and a cathode, producing thrust.
Hall thrusters offer a balance between efficiency and thrust, providing higher thrust levels than ion thrusters while maintaining good specific impulse. They are widely used for satellite station-keeping and orbit-raising operations.
Electrospray Propulsion
Electrospray propulsion, also known as colloid or field emission electric propulsion, uses electrostatic forces to accelerate charged droplets or ions from a liquid propellant. In this system, the propellant is typically an ionic liquid that is fed through a porous emitter. An electric field applied to the emitter extracts and accelerates the ions or droplets to produce thrust.
Electrospray propulsion systems are compact and offer precise thrust control, making them suitable for small satellites and fine attitude adjustments. Their high efficiency and scalability make them an attractive option for future space missions.
ESA’s Electric Propulsion Missions
Notable Missions Utilizing Electric Propulsion
The European Space Agency (ESA) has been a pioneer in adopting and advancing electric propulsion technologies. One of the most notable missions utilizing electric propulsion is the SMART-1 mission, launched in 2003. SMART-1 was equipped with a solar electric propulsion system, specifically an ion thruster, to travel to the moon. The mission demonstrated the feasibility of electric propulsion for interplanetary missions and provided valuable data for future exploration.
Another significant mission is the BepiColombo mission to Mercury, launched in 2018. BepiColombo employs a combination of chemical and electric propulsion, utilizing ion thrusters to conduct complex orbital maneuvers and reduce travel time. The mission aims to study Mercury’s surface and magnetic field, providing insights into the formation and evolution of the innermost planet in our solar system.
Success Stories and Milestones
ESA’s SMART-1 mission marked a major milestone in electric propulsion technology. The ion thruster used in SMART-1 allowed the spacecraft to achieve precise lunar orbit insertion and conduct scientific observations of the moon. The mission successfully demonstrated the long-term reliability and efficiency of electric propulsion in deep space environments.
The BepiColombo mission represents another success story for ESA’s electric propulsion efforts. The mission’s use of ion thrusters has enabled it to navigate the challenging environment around Mercury, conducting gravity assist maneuvers and precise trajectory corrections. This mission highlights the capability of electric propulsion to support complex and ambitious space exploration objectives.
These missions underscore ESA’s commitment to advancing electric propulsion technology and its potential to revolutionize space exploration. By leveraging the advantages of electric propulsion, ESA continues to push the boundaries of what is possible in space missions, paving the way for more efficient, sustainable, and far-reaching exploration of our solar system and beyond.
Nuclear Propulsion
Concept and Potential
Basics of Nuclear Propulsion
Nuclear propulsion involves using nuclear reactions to generate the thrust needed to propel spacecraft. Unlike traditional chemical propulsion, which relies on the combustion of fuel and oxidizer, nuclear propulsion uses either nuclear fission or nuclear fusion to produce a significant amount of energy. This energy is then used to heat a propellant, typically hydrogen, which is expelled at high velocities to create thrust.
There are two main types of nuclear propulsion: nuclear thermal propulsion (NTP) and nuclear electric propulsion (NEP). In NTP, a nuclear reactor heats a propellant directly, causing it to expand and be expelled through a nozzle to produce thrust. In NEP, a nuclear reactor generates electricity, which then powers an electric propulsion system such as an ion thruster.
Benefits and Risks
Nuclear propulsion offers several significant benefits for space exploration. The primary advantage is the high energy density of nuclear fuel, which allows for much greater efficiency and specific impulse compared to chemical propulsion. This means that spacecraft can travel farther and faster, making nuclear propulsion particularly advantageous for deep space missions and manned missions to distant planets like Mars.
Another benefit is the potential for sustained and continuous thrust, which can significantly reduce travel time to distant destinations. Nuclear propulsion can also provide the necessary power for onboard systems and instruments, ensuring that spacecraft can operate for extended periods in the harsh environment of space.
However, nuclear propulsion also comes with substantial risks and challenges. The most significant risk is the potential for radioactive contamination, both during launch and in space. Ensuring the safety of nuclear reactors and preventing the release of radioactive materials in the event of an accident is a critical concern. Additionally, the development and testing of nuclear propulsion systems require significant regulatory oversight and international cooperation to address environmental and safety concerns.
ESA’s Research and Development
Current Projects and Research Initiatives
The European Space Agency (ESA) is actively engaged in research and development of nuclear propulsion technologies, recognizing their potential to revolutionize space exploration. ESA collaborates with international partners, including NASA and Roscosmos, to explore the feasibility and application of nuclear propulsion for future missions.
One of ESA’s notable research initiatives is the assessment of nuclear thermal propulsion (NTP) for manned missions to Mars. This project involves studying the design and engineering challenges of developing a nuclear reactor suitable for space applications, as well as the potential benefits in terms of mission duration and payload capacity.
ESA is also exploring nuclear electric propulsion (NEP) for long-duration deep space missions. This research focuses on integrating nuclear reactors with advanced electric propulsion systems, such as ion thrusters, to provide a continuous and efficient thrust. The aim is to develop propulsion systems capable of supporting ambitious missions to the outer planets and beyond.
Future Plans and Potential Applications
Looking ahead, ESA’s future plans for nuclear propulsion include advancing the technology readiness levels of both NTP and NEP systems. This involves continued research, ground testing, and potential in-space demonstrations to validate the safety and performance of nuclear propulsion technologies.
Potential applications of nuclear propulsion extend beyond manned missions to Mars. For example, nuclear propulsion could enable faster and more efficient exploration of the outer planets, their moons, and even interstellar space. The high specific impulse and sustained thrust provided by nuclear propulsion would make it feasible to send scientific probes on long-duration missions to study the outer reaches of our solar system.
Moreover, nuclear propulsion could support the establishment of sustainable human presence on the Moon and Mars by providing reliable power and propulsion capabilities for habitat modules, cargo transports, and surface exploration vehicles.
ESA’s commitment to nuclear propulsion research and development underscores its dedication to advancing the frontiers of space exploration. By leveraging the unique advantages of nuclear propulsion, ESA aims to overcome the limitations of traditional propulsion methods and enable a new era of deep space exploration and scientific discovery.
Green Propulsion
Environmentally Friendly Propulsion Solutions
Definition and Importance
Environmentally friendly propulsion solutions, often referred to as green propulsion, involve the use of propellants and propulsion technologies that minimize harmful environmental impacts. Traditional propulsion systems, particularly those using toxic chemicals like hydrazine, pose significant risks to both the environment and human health. Green propulsion aims to address these concerns by developing alternatives that are safer, less toxic, and more sustainable.
The importance of green propulsion lies in its potential to reduce the environmental footprint of space missions. As the number of space missions increases, so does the need for sustainable practices to ensure that space exploration does not harm our planet. Green propulsion technologies contribute to this goal by minimizing the release of hazardous substances during the production, launch, and operation of spacecraft. Moreover, they enhance the safety of ground personnel and reduce the costs associated with handling and storing toxic propellants.
ESA’s Green Propulsion Projects
Examples of Green Propulsion Technologies
The European Space Agency (ESA) has been a leader in developing and promoting green propulsion technologies. ESA’s green propulsion projects focus on finding viable alternatives to traditional chemical propellants and improving the efficiency and sustainability of space missions.
One notable example is the development of propulsion systems that use ammonium dinitramide (ADN) as a propellant. ADN-based propellants are less toxic and more environmentally friendly compared to hydrazine. ESA has tested these propellants in various missions, demonstrating their feasibility and performance. For instance, the LISA Pathfinder mission employed an ADN-based propulsion system to control the spacecraft’s trajectory and perform precise maneuvers.
Another example is the use of hydrogen peroxide (H2O2) as a propellant. Hydrogen peroxide is a safer and less toxic alternative to traditional propellants and can be decomposed into water and oxygen, making it environmentally benign. ESA has explored the use of hydrogen peroxide in small satellite propulsion systems and launch vehicle upper stages.
ESA is also investigating the potential of hybrid propulsion systems that combine solid and liquid propellants. These systems offer the benefits of both propulsion types, such as the simplicity and reliability of solid propellants with the efficiency and controllability of liquid propellants. By optimizing the performance and reducing the environmental impact, hybrid propulsion systems represent a promising avenue for future green propulsion solutions.
Impact on the Environment and Mission Efficiency
Green propulsion technologies have a profound impact on both the environment and mission efficiency. By reducing the use of toxic propellants, these technologies minimize the risk of environmental contamination and protect ecosystems from harmful substances. This is particularly important for launch sites and spaceports, where the accumulation of toxic residues can have long-term environmental consequences.
In addition to environmental benefits, green propulsion technologies enhance mission efficiency. Non-toxic propellants simplify the handling and storage processes, reducing the operational costs and risks associated with traditional propellants. This can lead to significant cost savings for space missions, making them more economically viable.
Furthermore, green propulsion systems often provide higher specific impulse and better performance compared to their traditional counterparts. This means that spacecraft can achieve greater speeds and longer mission durations with the same amount of propellant. The improved efficiency translates to more ambitious and scientifically valuable missions, enabling deeper exploration of space and more comprehensive data collection.
Overall, ESA’s commitment to developing and implementing green propulsion technologies underscores the agency’s dedication to sustainable space exploration. By prioritizing environmental responsibility and operational efficiency, ESA is setting a benchmark for the future of space missions, ensuring that exploration beyond Earth does not come at the cost of our home planet.
Hybrid Propulsion
Combining Propulsion Technologies
Definition and Working Principles
Hybrid propulsion combines different propulsion technologies, typically solid and liquid propellants, to leverage the advantages of each. In a hybrid propulsion system, a solid propellant (usually the fuel) and a liquid or gaseous oxidizer are stored separately and only combine in the combustion chamber during operation. This setup allows the simplicity and reliability of solid propellants to be combined with the efficiency and controllability of liquid propellants.
The working principle of hybrid propulsion involves a solid fuel grain placed inside a combustion chamber, with the liquid or gaseous oxidizer stored in a separate tank. When the oxidizer is introduced into the combustion chamber, it reacts with the solid fuel to produce thrust. The rate of oxidizer flow can be controlled, allowing for throttle adjustments and the ability to stop and restart the engine. This provides greater flexibility and control compared to purely solid propulsion systems.
ESA’s Hybrid Propulsion Innovations
Recent Developments and Applications
The European Space Agency (ESA) has been at the forefront of developing hybrid propulsion technologies, recognizing their potential to enhance mission performance and sustainability. Recent developments have focused on optimizing the design and performance of hybrid engines, as well as exploring novel combinations of fuels and oxidizers.
One significant development is the use of paraffin-based fuels combined with liquid oxygen (LOX) as the oxidizer. Paraffin offers higher regression rates (the rate at which fuel is consumed) compared to traditional solid fuels, resulting in increased thrust levels. ESA’s research has demonstrated that paraffin/LOX hybrid engines can achieve high performance while maintaining the safety and environmental benefits of hybrid propulsion.
Another area of innovation is the use of nitrous oxide (N2O) as an oxidizer. Nitrous oxide is a non-toxic and stable oxidizer that simplifies handling and storage. ESA has been experimenting with various fuel combinations, including paraffin and hydroxyl-terminated polybutadiene (HTPB), to develop efficient and reliable hybrid propulsion systems using nitrous oxide.
Case Studies of Hybrid Propulsion Missions
ESA has conducted several missions and projects that showcase the application and benefits of hybrid propulsion technologies. One notable case study is the Hybrid Propulsion Demonstrator (HPD) project, which aimed to test and validate hybrid propulsion systems for small satellite launchers.
The HPD project involved a series of ground tests and flight demonstrations using a hybrid engine powered by HTPB fuel and liquid oxygen. The successful tests demonstrated the feasibility and performance of hybrid propulsion for small launch vehicles, highlighting its potential to provide cost-effective and flexible launch solutions for small satellites.
Another significant case study is the ESA-supported research into hybrid propulsion for lunar landers. The project explored the use of hybrid engines for descent and landing maneuvers, utilizing the controllability and efficiency of hybrid systems to achieve precise and safe lunar landings. The research findings indicated that hybrid propulsion could enhance the performance and safety of lunar missions, making it a viable option for future exploration endeavors.
Furthermore, ESA has collaborated with academic and industry partners to develop hybrid propulsion systems for sounding rockets. These rockets, used for suborbital research and technology testing, benefit from the throttleability and restart capability of hybrid engines, enabling more versatile and controlled flight profiles.
Solar Sails
Harnessing Solar Energy
Basic Principles of Solar Sails
Solar sails are a form of spacecraft propulsion that harnesses the energy of sunlight to generate thrust. The fundamental principle behind solar sails is the use of photons—particles of light—to push against a large, reflective sail. When sunlight strikes the sail, the momentum of the photons is transferred to the sail, creating a small but continuous force that propels the spacecraft.
Solar sails work on the principle of radiation pressure. Unlike traditional propulsion methods, which rely on expelling mass to generate thrust, solar sails utilize the momentum of photons to push against a large, lightweight sail material. The sail is typically made from a thin, reflective material, such as aluminized Mylar or Kapton, which maximizes the efficiency of photon momentum transfer.
The thrust generated by solar sails is low but constant, allowing spacecraft to gradually accelerate over time. This method of propulsion is particularly well-suited for long-duration missions in deep space, where the continuous thrust can build up substantial velocity over extended periods.
ESA’s Solar Sail Missions
Notable Projects and Achievements
ESA has been a pioneer in developing and deploying solar sail technology, with several notable projects showcasing the potential of this innovative propulsion method.
One of ESA’s landmark projects is the IKAROS (Interplanetary Kite-craft Accelerated by Radiation Of the Sun) mission, launched in 2010. IKAROS was the first spacecraft to successfully demonstrate solar sail propulsion in interplanetary space. The mission involved deploying a 14-meter-wide sail made from a thin, lightweight film. IKAROS successfully proved the viability of solar sails by demonstrating controlled propulsion and navigation using sunlight. The mission provided valuable data on sail deployment, stability, and performance, paving the way for future solar sail missions.
Another significant ESA project is the NEOSat mission, which includes a solar sail experiment designed to test and validate advanced sail technologies in space. The mission aims to explore new materials and designs for solar sails, as well as their performance in various space environments. NEOSat is expected to provide critical insights into the long-term behavior of solar sails and their potential applications for future space exploration.
Future Prospects and Planned Missions
ESA’s commitment to solar sail technology continues with ongoing research and future missions planned to further exploit the benefits of solar sails for space exploration. Future prospects include missions to explore the outer planets and beyond, where solar sails can provide a continuous and efficient propulsion solution.
One planned mission is the Solar Sail Demonstrator project, which aims to develop and test next-generation solar sail technologies. This mission will focus on improving the efficiency, durability, and deployment mechanisms of solar sails, with the goal of supporting more ambitious and long-duration space missions.
Additionally, ESA is collaborating with international partners to explore the use of solar sails for interstellar probes. These probes would use solar sails to gradually accelerate to a significant fraction of the speed of light, enabling exploration of the nearest star systems and expanding our understanding of the cosmos.
Advanced Propulsion Concepts
Breakthrough Theories and Ideas
Overview of Cutting-Edge Propulsion Concepts
The field of space propulsion is continuously evolving, driven by the need for more efficient, powerful, and versatile propulsion systems. Several cutting-edge propulsion concepts are currently being explored, each with the potential to revolutionize space travel and exploration.
Antimatter Propulsion
Antimatter propulsion is based on the principle that matter and antimatter annihilate each other upon contact, releasing energy according to Einstein’s equation E=mc2E=mc^2E=mc2. This reaction produces immense energy and could, theoretically, provide extremely high thrust and specific impulse. Although currently impractical due to the difficulty in producing and storing antimatter, research is ongoing to understand its potential applications for deep-space missions.
Nuclear Fusion Propulsion
Nuclear fusion propulsion aims to harness the energy produced by nuclear fusion reactions, similar to those occurring in the sun. Fusion reactions, such as those between isotopes of hydrogen, promise to provide vast amounts of energy with minimal radioactive byproducts. Concepts like fusion drives or “direct fusion” propulsion are being studied, which could potentially offer high thrust and efficiency for long-duration space travel.
Beamed Energy Propulsion
Beamed energy propulsion involves using external sources of energy, such as lasers or microwaves, beamed from Earth or a space-based platform, to propel a spacecraft. This method eliminates the need to carry propellant on board, relying instead on energy transmitted from a distance. Concepts such as the Laser Light Sail or the Breakthrough Starshot initiative aim to propel small spacecraft to nearby stars using powerful laser beams.
Quantum Propulsion
Quantum propulsion is a theoretical concept based on the manipulation of quantum effects to produce thrust. This includes ideas like the EmDrive, which claims to generate thrust without expelling propellant by exploiting quantum vacuum fluctuations. While highly speculative and controversial, such concepts are being explored for their potential to unlock new paradigms in propulsion technology.
ESA’s Exploration of Advanced Propulsion
Current Research and Experimental Projects
ESA is actively engaged in exploring advanced propulsion technologies to enhance the capabilities and efficiency of future space missions. The agency’s research spans various innovative concepts, including several that align with the cutting-edge theories mentioned above.
One notable project is the HELIOS (High Energy Laser for Interstellar Space) initiative, which investigates the feasibility of beamed energy propulsion. The project explores how high-energy lasers could be used to accelerate spacecraft to high speeds, enabling interstellar travel and advanced space exploration.
ESA is also involved in research related to nuclear fusion propulsion. The agency supports collaborative efforts with international partners to study fusion reactions and their potential applications in space propulsion. While practical fusion propulsion remains a long-term goal, ongoing research aims to advance our understanding and technological readiness for future developments.
Long-Term Vision and Goals
ESA’s long-term vision for advanced propulsion focuses on achieving breakthroughs that will enable more ambitious space missions, including crewed missions to Mars and beyond. The agency aims to develop propulsion systems that offer high efficiency, sustainability, and the capability to support long-duration exploration missions.
One key goal is to achieve practical and reliable nuclear fusion propulsion, which would revolutionize space travel by providing high energy density and efficient thrust. This would enable faster travel times to distant planets and support more extensive exploration of the solar system and beyond.
Another important goal is to advance beamed energy propulsion technologies, which could facilitate interstellar missions and open new frontiers in space exploration. By developing the necessary infrastructure and technology for beamed energy propulsion, ESA envisions a future where spacecraft can reach other star systems and expand our knowledge of the universe.
ESA’s commitment to exploring breakthrough theories and ideas reflects its dedication to pushing the boundaries of space technology and achieving new milestones in space exploration. By investing in cutting-edge research and experimental projects, ESA aims to transform the possibilities of space travel and make previously unimaginable missions a reality.
Propulsion Technology and Satellite Deployment
Enhancing Satellite Operations
Importance of Propulsion in Satellite Missions
Propulsion systems play a crucial role in the operation and performance of satellites. Unlike spacecraft designed for deep space exploration, satellites typically operate in Earth’s orbit and are subject to various dynamic forces, including gravitational perturbations and atmospheric drag. Effective propulsion is essential for:
Orbit Insertion and Maintenance
Propulsion systems enable satellites to reach and maintain their designated orbits after launch. Accurate orbit insertion is vital for positioning satellites in their intended operational paths, whether for communication, observation, or scientific purposes. Once in orbit, propulsion systems can perform maneuvers to maintain the satellite’s position and counteract perturbations from gravitational forces or collisions with space debris.
Attitude Control
Satellite attitude control involves adjusting the satellite’s orientation to ensure that its instruments or antennas are correctly aligned with their targets. Propulsion systems, including thrusters and reaction wheels, are used to achieve precise attitude adjustments. This capability is crucial for missions requiring high-resolution imaging, data collection, or communication with ground stations.
End-of-Life Deorbiting
At the end of their operational life, satellites must be deorbited safely to minimize space debris. Propulsion systems are used to execute controlled deorbiting maneuvers, ensuring that satellites re-enter Earth’s atmosphere and burn up before posing any risk to operational satellites or space stations.
ESA’s Satellite Propulsion Technologies
Innovations and Their Impact on Satellite Performance
The European Space Agency (ESA) has developed and implemented several advanced propulsion technologies to enhance satellite performance and mission capabilities. These innovations reflect ESA’s commitment to improving satellite operations through efficient and reliable propulsion systems.
Electric Propulsion
ESA has made significant advancements in electric propulsion, particularly with ion thrusters and Hall effect thrusters. Electric propulsion systems are known for their high efficiency and specific impulse, making them ideal for long-duration missions and precise orbital adjustments.
For example, ESA’s SMART-1 lunar mission employed an ion thruster for propulsion, demonstrating the capability of electric propulsion to achieve efficient orbit insertion and precise trajectory control. The high efficiency of electric propulsion allows satellites to carry less propellant, freeing up space for additional scientific instruments or payloads.
Green Propulsion
ESA is also at the forefront of developing environmentally friendly propulsion technologies, such as green propellants that replace traditional toxic chemicals. These green propellants, such as those based on ammonium dinitramide (ADN), offer safer handling and reduced environmental impact.
Green propulsion technologies improve satellite performance by enabling more flexible and efficient propulsion solutions. For instance, ESA’s work on ADN-based propulsion has shown promise in enhancing satellite maneuverability and extending mission lifetimes while minimizing the environmental footprint.
Hybrid Propulsion
ESA is exploring hybrid propulsion systems that combine solid and liquid propellants. These systems offer the advantages of both propulsion types, including higher thrust levels and controllability. Hybrid propulsion provides a versatile solution for various satellite missions, from launch vehicle upper stages to attitude control systems.
The development of hybrid propulsion technologies has the potential to improve satellite performance by offering more efficient and reliable propulsion options. Hybrid systems can enhance maneuverability, optimize fuel usage, and extend operational lifetimes.
Chemical Propulsion Innovations
ESA continues to advance traditional chemical propulsion technologies, refining existing systems to enhance performance and reliability. Innovations include improved thruster designs and more efficient propellant formulations, which contribute to better satellite operations.
For example, ESA’s work on optimized chemical thrusters has led to improvements in thrust control and reliability, ensuring that satellites can perform precise maneuvers and maintain optimal operational configurations throughout their mission lifetimes.
Propulsion in Interplanetary Missions
Enabling Deep Space Exploration
Role of Propulsion Technologies in Interplanetary Travel
Propulsion technologies are pivotal for interplanetary travel, as they determine a spacecraft’s ability to reach, navigate, and operate within different planetary environments. For missions beyond Earth’s orbit, propulsion systems must provide sufficient thrust to escape Earth’s gravity and maintain the required velocity to travel vast distances through space. Efficient propulsion also supports trajectory corrections, orbital insertions, and rendezvous with target celestial bodies. Advanced propulsion technologies, including electric, chemical, and potentially nuclear systems, enable spacecraft to achieve the high speeds necessary for interplanetary travel, manage long-duration missions, and ensure the successful execution of complex scientific objectives.
ESA’s Interplanetary Mission Propulsion
The European Space Agency (ESA) has been instrumental in advancing propulsion technologies for interplanetary exploration. ESA’s interplanetary missions have utilized a range of propulsion systems tailored to the specific requirements of each mission. For instance, ESA’s SMART-1 lunar mission employed an ion propulsion system, which provided efficient and sustained thrust over an extended period, allowing the spacecraft to reach lunar orbit with minimal propellant. Similarly, the Rosetta mission to Comet 67P/Churyumov-Gerasimenko utilized a combination of chemical propulsion for initial trajectory adjustments and gravity assists, complemented by fine-tuning maneuvers using its onboard propulsion system to achieve precise orbital control. These missions demonstrate ESA’s capability to leverage advanced propulsion methods to achieve ambitious interplanetary goals.
Key Missions and Propulsion Methods Used
ESA has undertaken several notable interplanetary missions, each utilizing specific propulsion methods to achieve its objectives. The SMART-1 mission, launched in 2003, was a pioneering project that utilized a solar-powered ion propulsion system to explore the Moon. This mission highlighted the efficiency of electric propulsion for long-duration space missions. The Rosetta mission, launched in 2004, employed a mix of chemical propulsion and gravity assists to travel to Comet 67P, where it successfully deployed the Philae lander on the comet’s surface. Another key mission, ExoMars, employed a combination of chemical propulsion for its descent and landing phases, and precision thrusters for orbit insertion and fine-tuning maneuvers. These missions exemplify the diverse propulsion strategies ESA uses to address the unique challenges of interplanetary exploration.
Success Stories and Learnings
ESA’s interplanetary missions have yielded significant successes and valuable insights into the role of propulsion technologies. The SMART-1 mission demonstrated the effectiveness of ion propulsion for deep space exploration, providing crucial data on the lunar surface and paving the way for future missions using similar technology. The Rosetta mission was a landmark achievement, successfully orbiting and landing on a comet, thereby expanding our understanding of these distant objects and showcasing the capabilities of a hybrid propulsion approach. The ExoMars mission provided important lessons in landing precision and atmospheric entry techniques, improving future mission planning and execution. These successes underscore the importance of innovative propulsion technologies in enabling deep space exploration and achieving scientific breakthroughs.
Human Spaceflight Propulsion
Ensuring Safe and Efficient Travel
Specific Needs of Human Spaceflight Propulsion
Human spaceflight requires propulsion systems that address unique challenges beyond those faced in unmanned missions. Key needs include reliable and robust systems capable of supporting crewed spacecraft during launch, in-space maneuvers, and re-entry. Propulsion systems must ensure safe and precise trajectory control to avoid potential hazards and ensure accurate docking with space stations or other spacecraft. Additionally, they must be capable of providing adequate thrust for landing operations and orbital adjustments while minimizing risks to the crew. Systems must also be designed to handle the long-duration missions’ requirements, such as maintaining life-support systems and managing spacecraft resources efficiently.
ESA’s Contributions to Human Spaceflight Propulsion
The European Space Agency (ESA) has made significant contributions to the development of propulsion systems for human spaceflight, focusing on safety, efficiency, and reliability. ESA’s involvement in human spaceflight includes collaboration on the development of propulsion systems used in various crewed missions, such as those involving the International Space Station (ISS) and future deep space exploration. ESA has contributed to the design and enhancement of propulsion systems for spacecraft like the Automated Transfer Vehicle (ATV) and the Orion crew module, ensuring they meet the rigorous requirements for crew safety and mission success.
ESA’s work on the ATV, for example, involved developing reliable propulsion systems for docking with the ISS and performing necessary orbit adjustments. The ATV utilized a combination of chemical propulsion systems for precise docking maneuvers and trajectory corrections, demonstrating ESA’s commitment to ensuring safe and efficient travel for astronauts. Additionally, ESA is actively involved in the development of advanced propulsion technologies that will support future crewed missions to the Moon and Mars, including innovative concepts that promise enhanced performance and safety for long-duration human spaceflight.
Projects and Technologies Developed for Human Missions
ESA has developed and supported several key projects and technologies specifically designed for human spaceflight. One prominent example is the development of the Ariane 5 rocket, which has been instrumental in launching both crewed and uncrewed missions. The Ariane 5’s propulsion systems are designed to provide reliable and powerful thrust, ensuring the successful deployment of payloads into orbit and supporting ESA’s crewed missions.
Another significant project is the ExoMars program, which includes the development of propulsion systems for future crewed Mars missions. The program focuses on creating technologies that will ensure safe transit to Mars, efficient orbital insertion, and successful surface operations. ESA’s research into hybrid and electric propulsion systems also aims to improve the performance and safety of future crewed spacecraft, providing better efficiency and reliability for long-duration missions.
ESA’s contributions extend to international collaborations, such as those with NASA on the Orion crew module, where ESA has provided critical propulsion technologies for deep space exploration. These collaborations help to integrate advanced propulsion systems into crewed spacecraft, ensuring they meet the high standards required for human spaceflight.
Overall, ESA’s advancements in propulsion technologies reflect a commitment to enhancing the safety, efficiency, and reliability of human spaceflight. By developing and implementing cutting-edge technologies, ESA supports successful crewed missions and contributes to the broader goals of space exploration and scientific discovery.
Propulsion and Space Debris Management
Addressing the Growing Space Debris Problem
Role of Propulsion in Managing Space Debris
Propulsion systems are essential in addressing the growing issue of space debris, which poses significant risks to operational satellites and spacecraft. Effective management of space debris involves a combination of strategies, including active debris removal and collision avoidance. Propulsion technologies play a key role in these strategies by enabling spacecraft to perform collision avoidance maneuvers and execute controlled deorbiting operations. By adjusting the orbits of satellites and decommissioned spacecraft, propulsion systems can prevent potential collisions and reduce the accumulation of space debris in critical orbits. Additionally, propulsion systems are integral to active debris removal missions, which aim to capture and safely remove defunct satellites and debris from orbit.
ESA’s Initiatives for Space Debris Mitigation
The European Space Agency (ESA) has been proactive in developing and implementing initiatives to mitigate the space debris problem. ESA’s approach includes a combination of policy development, technology innovation, and collaborative efforts with international partners. One of ESA’s key initiatives is the development of the Space Debris Mitigation Guidelines, which provide best practices and technical recommendations for satellite operators to reduce the creation of new debris. These guidelines emphasize measures such as end-of-life disposal plans, the use of non-rocket methods for orbit raising and lowering, and the design of satellites to minimize debris generation.
ESA has also led several projects focused on debris removal and management. One notable project is the ClearSpace-1 mission, which aims to demonstrate and operationalize technologies for capturing and removing space debris. Scheduled for launch in the mid-2020s, ClearSpace-1 will use a robotic arm to capture a defunct piece of space debris and guide it into a lower orbit, where it will eventually re-enter the Earth’s atmosphere and burn up. This mission represents a significant step towards developing practical solutions for active debris removal.
Technologies and Strategies Implemented
ESA has developed and implemented several technologies and strategies to address space debris, reflecting its commitment to ensuring the long-term sustainability of space activities. Among these technologies, the use of propulsion systems for collision avoidance is a primary strategy. ESA supports the implementation of collision avoidance maneuvers for its satellites and encourages other space agencies and operators to adopt similar practices. This involves real-time tracking of space debris and predictive modeling to assess potential collision risks, followed by propulsion-based adjustments to satellite trajectories.
For debris removal, ESA has explored various technological approaches, including electrodynamic tethers and robotic capture systems. Electrodynamic tethers generate thrust through interactions with the Earth’s magnetic field, which can be used to lower the orbits of defunct satellites and accelerate their decay. Robotic capture systems, as demonstrated in the ClearSpace-1 mission, involve capturing debris using mechanical arms or nets and guiding it to a controlled re-entry.
ESA also invests in research to develop new materials and design techniques that reduce the creation of debris from new satellites. These include measures such as using non-explosive separation systems, designing satellites to withstand space impacts without generating fragments, and implementing de-orbiting plans that ensure satellites are removed from orbit at the end of their operational life.
Overall, ESA’s initiatives and strategies reflect a comprehensive approach to managing the space debris problem, leveraging advanced propulsion technologies, innovative removal techniques, and proactive policy development to safeguard space operations and ensure the sustainable use of space.
Collaboration and Partnerships
Working with International Space Agencies
Importance of Collaboration in Propulsion Technology Development
Collaboration among international space agencies is crucial for advancing propulsion technology, as it enables the sharing of expertise, resources, and innovative ideas. Developing advanced propulsion systems often requires substantial investment and research capabilities that go beyond the scope of any single organization. By working together, space agencies can pool their resources to tackle complex challenges, accelerate technological advancements, and achieve shared goals more efficiently. International partnerships also facilitate the exchange of knowledge and best practices, leading to the development of more effective and versatile propulsion technologies. Additionally, collaborative efforts help to standardize technologies and protocols, which is essential for interoperability and the success of joint missions.
ESA’s Key Partnerships and Collaborations
The European Space Agency (ESA) has established numerous key partnerships and collaborations with space agencies and organizations worldwide to advance propulsion technology. These partnerships enhance ESA’s capabilities and contribute to the global space exploration community. One of ESA’s primary international collaborators is NASA, with whom ESA has worked on several joint projects involving propulsion systems. Another significant partner is the Russian space agency, Roscosmos, which collaborates with ESA on various space missions and technology development initiatives. ESA also engages in partnerships with the Japanese Aerospace Exploration Agency (JAXA) and the Canadian Space Agency (CSA), among others, to support a wide range of space missions and technological advancements.
Notable Joint Projects and Their Outcomes
One of the most notable joint projects involving ESA is the International Space Station (ISS), a collaborative effort with NASA, Roscosmos, JAXA, and the Canadian Space Agency. The ISS relies on various propulsion technologies to maintain its orbit, perform attitude control, and support docking operations. ESA contributes to the ISS through its European Automated Transfer Vehicle (ATV) program, which has demonstrated advanced propulsion systems for cargo delivery and orbit adjustments. The ATV’s successful missions have provided valuable insights into propulsion technology and its applications in space station logistics.
Another significant collaborative project is the ExoMars program, a joint effort between ESA and Roscosmos. This program includes the ExoMars Trace Gas Orbiter and the ExoMars Rover, which aim to explore Mars and search for signs of past or present life. The propulsion systems used in the ExoMars missions involve both ESA and Roscosmos technologies, highlighting the integration of different propulsion approaches to achieve mission objectives. The successful deployment and operation of these systems have advanced our understanding of Martian geology and atmospheric composition.
ESA’s collaboration with NASA on the Orion crew module is another example of successful international cooperation. The Orion spacecraft, designed for deep space exploration, benefits from ESA’s contributions to its propulsion systems, including the European Service Module, which provides critical propulsion and power systems for the spacecraft. This collaboration has demonstrated the effectiveness of integrating international technologies to support human spaceflight and exploration beyond low Earth orbit.
Future Trends in Propulsion Technologies
Anticipated Innovations and Developments
Emerging Trends and Technologies in Propulsion
The field of space propulsion is on the cusp of several exciting innovations and technological advancements. Emerging trends are focused on improving efficiency, reducing costs, and expanding the possibilities of space travel. One significant trend is the development of advanced electric propulsion systems, including next-generation ion thrusters and Hall effect thrusters, which offer higher efficiency and longer mission durations. These technologies are expected to enhance capabilities for deep space exploration and satellite maneuvering.
Another promising area is the exploration of nuclear propulsion, which could revolutionize space travel by providing high energy density and thrust. Innovations in nuclear thermal and nuclear electric propulsion are being investigated to enable faster transit times to distant planets and support long-term human missions. Additionally, solar sails and beamed energy propulsion represent groundbreaking approaches to harnessing solar energy and external power sources for propulsion, potentially allowing spacecraft to travel at unprecedented speeds.
On the front of green and sustainable technologies, researchers are working on environmentally friendly propellants and propulsion systems that minimize the impact on the space environment. This includes developing new materials and techniques that reduce the generation of space debris and enhance the longevity and safety of space missions.
ESA’s Roadmap for Future Propulsion Technologies
ESA has developed a comprehensive roadmap to guide the future of propulsion technologies, aligning with its strategic goals and mission objectives. This roadmap outlines key areas of focus, including the advancement of current technologies and the exploration of novel concepts to address future space exploration needs.
Strategic Goals and Upcoming Projects
ESA’s strategic goals for future propulsion technologies include increasing mission efficiency, expanding exploration capabilities, and ensuring sustainable practices. One major focus is the development of next-generation electric propulsion systems to support long-duration missions and complex satellite operations. The agency aims to enhance the performance of ion and Hall effect thrusters and integrate these systems into a broader range of missions.
ESA is also investing in research and development for nuclear propulsion technologies, with the goal of enabling faster and more efficient travel to distant destinations such as Mars and beyond. Projects like the Nuclear Electric Propulsion (NEP) initiative are exploring how nuclear energy can be harnessed to provide high thrust and extended mission capabilities.
Another critical aspect of ESA’s roadmap is the advancement of green propulsion technologies. The agency is working on the development of new propellants and systems that reduce environmental impact and improve safety. The Green Propellant program, for example, focuses on replacing traditional toxic propellants with safer, more sustainable alternatives.
ESA’s upcoming projects include the ClearSpace-1 mission, which aims to demonstrate active debris removal technologies. This project represents a significant step towards addressing the issue of space debris and ensuring the long-term sustainability of space operations
Conclusion
Summary of Key Points
Innovative propulsion technologies play a crucial role in advancing space exploration by enhancing the efficiency, capability, and sustainability of space missions. Traditional chemical propulsion has served as the foundation for space travel, but recent advancements in electric, nuclear, and green propulsion technologies are transforming the landscape. Electric propulsion systems, such as ion and Hall effect thrusters, offer high efficiency and are particularly suited for long-duration missions and precise maneuvering. Nuclear propulsion holds the promise of enabling faster travel to distant planets by providing high energy density. Green propulsion technologies focus on reducing the environmental impact of space operations through safer and more sustainable propellants.
ESA’s contributions to propulsion technology include significant advancements and collaborations with international space agencies. Notable projects like the SMART-1, Rosetta, and ExoMars missions showcase the application of these technologies in real-world scenarios, demonstrating their effectiveness and potential. ESA’s ongoing and future projects, such as the ClearSpace-1 mission and developments in nuclear propulsion, highlight the agency’s commitment to addressing current challenges and exploring new possibilities in space travel.
The Impact of Innovative Propulsion Technologies on Space Exploration
Innovative propulsion technologies have a profound impact on space exploration by expanding the scope of missions, improving efficiency, and enhancing safety. These technologies enable spacecraft to achieve higher speeds, reach distant destinations more quickly, and conduct complex operations with greater precision. Electric propulsion systems, for example, provide significant benefits for deep space missions by allowing spacecraft to operate for extended periods with reduced propellant requirements. This capability enhances mission longevity and allows for more ambitious exploration objectives.
Nuclear propulsion offers the potential for revolutionary advancements in space travel by reducing transit times to distant planets and supporting long-duration human missions. The increased thrust and energy density provided by nuclear propulsion systems could enable faster and more efficient exploration of the outer planets and beyond, opening new frontiers in space science and exploration.
Green propulsion technologies contribute to the sustainability of space operations by minimizing the environmental impact of space missions. By developing and implementing safer and more sustainable propellants, space agencies can reduce the generation of space debris and enhance the safety of space environments for future missions.
FAQs
What is the most significant propulsion technology developed by ESA?
One of the most significant propulsion technologies developed by the European Space Agency (ESA) is electric propulsion, particularly ion thrusters. ESA’s work in this field includes the development and deployment of the SMART-1 lunar mission, which utilized an ion propulsion system to reach lunar orbit. The success of this mission demonstrated the high efficiency and long-duration capabilities of electric propulsion, marking a pivotal advancement in space technology. Electric propulsion systems, such as those developed by ESA, offer higher specific impulse compared to traditional chemical propulsion, allowing spacecraft to achieve significant orbital changes with less propellant. This technology represents a major leap forward in enabling more ambitious and cost-effective space missions.
How does electric propulsion differ from traditional chemical propulsion?
Electric propulsion differs from traditional chemical propulsion primarily in its efficiency and method of generating thrust. Traditional chemical propulsion relies on the combustion of propellants to produce high-pressure gases that are expelled to create thrust. This method is known for its high thrust but relatively lower specific impulse, meaning it requires more propellant for a given amount of thrust and results in higher fuel consumption.
In contrast, electric propulsion systems, such as ion thrusters and Hall effect thrusters, use electrical energy to ionize and accelerate propellant particles to generate thrust. These systems offer much higher specific impulse, which translates to more efficient use of propellant and the ability to achieve greater speeds over extended periods. While electric propulsion typically provides lower thrust compared to chemical propulsion, its efficiency makes it ideal for long-duration missions and precise maneuvers. This efficiency allows spacecraft to operate for longer periods with less propellant, making it a valuable technology for deep space exploration.
What are the environmental benefits of green propulsion?
Green propulsion technologies are designed to minimize the environmental impact of space missions by using safer, more sustainable propellants. The environmental benefits of green propulsion include:
- Reduced Toxicity: Green propellants are generally less harmful to humans and the environment compared to traditional chemical propellants. For example, green propellants like those based on ammonium dinitramide (ADN) have lower toxicity and are easier to handle, reducing the risk of accidents during storage and transportation.
- Lower Space Debris Generation: Some green propulsion technologies aim to reduce the amount of space debris generated by spacecraft. By minimizing explosive propellant residues and designing systems to deorbit defunct satellites more effectively, green propulsion contributes to reducing the accumulation of debris in orbit.
- Improved Sustainability: Green propulsion supports the long-term sustainability of space activities by focusing on propellants that are less likely to contribute to environmental contamination in space. This approach aligns with the broader goal of maintaining a cleaner and safer space environment for future missions.
What future propulsion technologies is ESA exploring?
ESA is exploring several advanced propulsion technologies to support future space exploration missions. Some key areas of focus include:
- Nuclear Propulsion: ESA is investigating nuclear thermal and nuclear electric propulsion systems, which promise to offer high energy density and thrust for deep space missions. These technologies could significantly reduce travel times to distant planets and support long-duration crewed missions.
- Advanced Electric Propulsion: ESA continues to develop next-generation electric propulsion systems, including improvements to ion and Hall effect thrusters. These advancements aim to enhance efficiency, increase thrust, and expand the capabilities of electric propulsion for various mission types.
- Green Propulsion Innovations: ESA is working on further advancements in green propulsion technologies to improve sustainability and reduce environmental impact. This includes developing new, less toxic propellants and systems designed to minimize space debris and ensure safer space operations.
- Solar Sails: ESA is exploring the use of solar sails, which utilize the pressure of solar radiation for propulsion. This technology has the potential to provide continuous thrust for extended missions, allowing spacecraft to travel long distances using only solar energy.