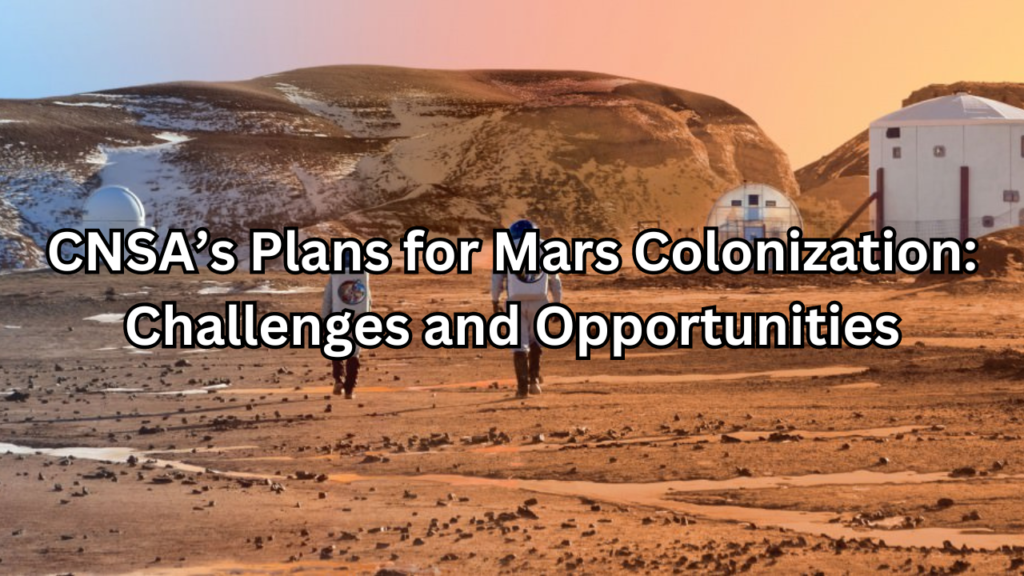
Table of Contents
Introduction
Overview of CNSA Plans for Mars Colonization Ambitions
The China National Space Administration (CNSA) has set ambitious goals for its space exploration program, with CNSA Plans for Mars Colonization emerging as a central component of its long-term vision. This bold objective underscores China’s commitment to becoming a leading player in the space industry, pushing the boundaries of human spaceflight, and ensuring its position at the forefront of extraterrestrial exploration. CNSA’s Mars colonization plans are not merely about reaching the Red Planet but envision a future where humans can establish a sustainable presence, conduct scientific research, and eventually expand human civilization beyond Earth.
Brief History of CNSA’s Space Exploration
China’s space journey began in the early 1950s, but significant milestones have been achieved since the establishment of CNSA in 1993. Over the decades, CNSA has progressed from launching its first satellite, Dong Fang Hong 1, in 1970, to developing its own manned spaceflight program, Tiangong space station, and successful Mars missions. The Chang’e lunar exploration program has been instrumental in enhancing China’s space capabilities, providing valuable experience in deep space exploration. The successful landing of the Tianwen-1 rover on Mars in 2021 marked a significant achievement, showcasing China’s growing prowess in space technology and its readiness to tackle the challenges of Mars colonization.
Importance of CNSA Plans for Mars Colonization in China’s Space Strategy
CNSA Plans for Mars Colonization is a pivotal element in China’s space strategy for several reasons. Firstly, it represents a major step in the quest for space exploration and scientific discovery. By establishing a presence on Mars, China aims to contribute to our understanding of the planet’s geology, climate, and potential for supporting life. Additionally, Mars serves as a crucial testing ground for technologies and systems that will be vital for future deep-space missions, including crewed missions to other celestial bodies.
Economically and strategically, Mars colonization positions China as a leading space power, enhancing its global influence and technological leadership. It also offers potential opportunities for resource utilization, including the extraction of minerals and elements that could be valuable for both space and terrestrial applications. Moreover, achieving Mars colonization would symbolize a significant milestone in human exploration, reflecting China’s growing capabilities and ambition on the global stage.
The Vision for Mars Colonization
Long-term Goals and Milestones
CNSA Plans for Mars Colonization is driven by several ambitious long-term goals, each designed to advance human capabilities in space and establish a sustainable presence on the Red Planet. At the heart of this vision is a commitment to scientific exploration, aimed at unlocking the secrets of Mars’ geology, climate, and potential for past or present life. CNSA plans to deploy advanced rovers and landers equipped with cutting-edge scientific instruments to gather vital data and conduct experiments that could revolutionize our understanding of Mars.
Technological innovation is another crucial component of CNSA’s vision. The development of technologies for long-term habitation, such as advanced life support systems and habitat construction methods, is essential for establishing a permanent human presence. CNSA aims to create environments that support human life, ensuring sustainable food and water supplies, and addressing health and safety concerns.
In addition to scientific and technological goals, CNSA envisions utilizing Martian resources to support human activities. This involves developing in-situ resource utilization (ISRU) technologies to extract water and minerals, thereby reducing dependency on Earth-based supplies. The vision also includes fostering international collaboration to enhance the success and sustainability of Mars missions, leveraging the expertise and resources of global partners to achieve common goals.
Timeline and Key Phases
CNSA Plans for Mars Colonization is structured into several key phases, each marking significant milestones in the journey towards establishing a human presence on Mars. The initial phase, spanning from 2020 to 2030, focuses on preliminary research and robotic missions. This includes deploying robotic explorers like the Tianwen-1 mission, which successfully landed the Zhurong rover on Mars in 2021. These early missions are crucial for gathering data on Mars’ surface and environment, laying the groundwork for future endeavors.
The next phase, from 2030 to 2040, involves advanced robotic exploration and technology validation. During this period, CNSA plans to deploy more sophisticated rovers and landers to conduct detailed geological surveys and identify potential landing sites for human missions. Additionally, habitat prototypes and life support systems will be tested in simulated Martian conditions to ensure readiness for crewed missions.
Between 2040 and 2050, CNSA aims to initiate crewed missions to Mars and establish an initial base. This phase focuses on setting up habitats, conducting scientific research, and testing in-situ resource utilization technologies. The construction of a permanent base on Mars will begin, emphasizing habitat stability and environmental control systems to support human life.
Technological Challenges
Spacecraft Design and Development
Innovations in Spacecraft Technology
Spacecraft design for Mars missions involves cutting-edge innovations aimed at addressing the unique challenges of interplanetary travel. One significant advancement is the development of advanced propulsion systems, such as nuclear thermal and electric propulsion, which offer greater efficiency and reduced travel time compared to traditional chemical rockets. These propulsion technologies are critical for overcoming the vast distance between Earth and Mars and for ensuring that spacecraft can carry sufficient payloads, including crew and scientific equipment.
Another major innovation is the integration of autonomous systems and artificial intelligence (AI) for navigation and operations. Autonomous systems allow spacecraft to perform complex tasks without real-time input from Earth, such as adjusting trajectories, managing resources, and conducting scientific experiments. AI enhances these capabilities by providing adaptive decision-making and problem-solving skills, which are crucial for handling unexpected challenges during long-duration missions.
Additionally, advancements in materials science and engineering have led to the development of lightweight, durable materials that can withstand the harsh environment of space and the Martian atmosphere. These materials not only improve the spacecraft’s structural integrity but also contribute to better thermal management and radiation protection, ensuring the safety and reliability of the spacecraft throughout its journey and mission.
Key Design Considerations for Mars Missions
Designing spacecraft for Mars missions requires addressing several critical considerations to ensure successful operations and crew safety. One primary consideration is the spacecraft’s ability to handle the vast distance and prolonged travel time to Mars. This involves designing propulsion systems that provide efficient and reliable thrust, as well as incorporating systems for deep-space navigation and communication.
Radiation protection is another crucial factor. Mars missions expose spacecraft and crew to higher levels of cosmic radiation and solar particles compared to missions within Earth’s orbit. Therefore, spacecraft must be equipped with effective radiation shielding to protect both the vehicle and its occupants from harmful radiation, which could otherwise lead to health risks and equipment malfunctions.
Life support systems are essential for maintaining a habitable environment inside the spacecraft. These systems must ensure a continuous supply of oxygen, manage carbon dioxide levels, and provide water and food. Additionally, the spacecraft must include systems for waste management and environmental control to support long-duration missions and sustain human life.
Propulsion Systems and Fuel Efficiency
Advances in Propulsion Technology
Advances in propulsion technology are pivotal for the success of Mars missions, addressing the challenges of long-duration space travel. One significant advancement is the development of nuclear thermal propulsion (NTP) systems. NTP uses nuclear reactors to heat a propellant, typically hydrogen, to high temperatures, creating a high-efficiency thrust. This technology offers a substantial increase in performance over conventional chemical rockets, potentially reducing travel time to Mars by several months.
Another promising technology is electric propulsion, which includes ion and Hall effect thrusters. These systems use electrical energy to ionize and accelerate a propellant, such as xenon, creating a high-speed exhaust stream. Electric propulsion is known for its high efficiency and capability for long-duration missions, though it produces lower thrust compared to chemical propulsion. Its efficiency is particularly advantageous for deep-space missions where long-term thrust is required.
Recent developments also include advances in chemical propulsion, such as high-efficiency bi-propellant engines that combine fuels and oxidizers to enhance performance and reduce waste. Innovations in engine design and fuel formulations are improving the efficiency and reliability of traditional rocket systems, making them more suitable for Mars missions.
Fuel Requirements and Sustainability
Fuel requirements for Mars missions are substantial, given the need for both propulsion and in-situ resource utilization. For interplanetary travel, spacecraft need large quantities of fuel to achieve escape velocity and sustain the journey to Mars. Efficient fuel usage and management are critical to ensure that spacecraft can carry enough propellant for both the outbound journey and return trip, if applicable.
Sustainability is also a key consideration. Utilizing in-situ resources on Mars, such as extracting water from the Martian soil and atmosphere to produce fuel, could significantly reduce the amount of fuel that needs to be launched from Earth. Technologies for producing fuel on Mars, such as the Mars Oxygen In-Situ Resource Utilization Experiment (MOXIE), aim to convert Martian carbon dioxide into oxygen and methane, which can be used for propulsion and life support.
Developing efficient fuel storage and management systems is crucial to maintaining fuel integrity over the extended duration of CNSA Space Missions. Innovations in fuel storage technologies, such as cryogenic tanks and advanced composites, help minimize fuel loss and ensure reliable delivery throughout the mission.
Landing and Ascent Technologies
Challenges of Landing on Mars
Landing on Mars presents unique challenges due to its thin atmosphere, varying terrain, and harsh environmental conditions. Unlike Earth, Mars has an atmosphere that is too thin to provide significant aerodynamic drag, making traditional parachute landing techniques less effective. The thin atmosphere also increases the risk of high-speed impacts with the surface, requiring advanced landing systems to ensure a safe touchdown.
The variability of Martian terrain poses additional difficulties. Mars has diverse surface features, including rugged mountains, deep valleys, and vast plains. Designing landing systems that can adapt to various terrains and ensure precise landings is essential to avoid hazardous locations and ensure mission success.
Another challenge is the need for robust thermal protection during entry and descent. The spacecraft must withstand intense heat generated by atmospheric friction during the entry phase, requiring advanced heat shield materials and design to protect the vehicle and its payload.
Technologies for Safe Landing and Ascent
To overcome the challenges of landing on Mars, several advanced technologies are employed. One key technology is the use of advanced heat shields, such as ablative materials that absorb and dissipate heat during atmospheric entry. These shields are designed to withstand extreme temperatures and protect the spacecraft during descent.
Precision landing systems are also crucial. Techniques such as Terrain-Relative Navigation (TRN) use onboard sensors and cameras to assess the landing site in real-time, allowing the spacecraft to adjust its descent trajectory and land accurately on the chosen site. This technology helps avoid hazardous terrain and ensures a safe landing.
For ascent from the Martian surface, technologies such as ascent rockets and aerodynamic lift systems are utilized. Mars ascent vehicles are designed to launch from the planet’s surface, overcoming the lower gravity and thin atmosphere. These rockets must be equipped with powerful engines and efficient propulsion systems to achieve escape velocity and return to orbit.
Habitat Construction and Sustainability
Designing Habitats for Mars Environment
Designing habitats for the Martian environment involves addressing the planet’s extreme conditions and ensuring that the living spaces are suitable for human life. Key design considerations include protecting against radiation, managing temperature extremes, and providing adequate life support systems. Mars habitats must be shielded from cosmic radiation and solar particles, which pose significant health risks. Radiation protection can be achieved through thick walls made of regolith or advanced composite materials.
Temperature control is another critical aspect. Mars experiences wide temperature fluctuations, ranging from extremely cold nights to relatively warmer daytime temperatures. Designing habitats with effective thermal insulation and climate control systems ensures that the interior remains stable and comfortable for inhabitants.
Air and water management are essential for sustaining life. Habitats must include systems for air purification, oxygen generation, and water recycling. Advanced life support systems will provide a continuous supply of breathable air and potable water, supporting long-term human missions.
Building Materials and Construction Methods
Building materials and construction methods for Martian habitats must be adapted to the planet’s unique conditions. One approach is to utilize Martian regolith, the loose surface material on Mars, as a primary building material. Regolith can be processed and used to create durable bricks or concrete-like substances, reducing the need to transport materials from Earth.
In-situ construction techniques, such as 3D printing, are being explored for building habitats on Mars. 3D printers can use local materials to construct habitat components layer by layer, minimizing the need for pre-fabricated parts and reducing the overall payload. This method also allows for flexible and scalable habitat design, adapting to different mission requirements.
Inflatable habitats are another innovative approach. These structures can be launched compactly and expanded once on Mars, providing a larger living space while minimizing launch volume. Inflatable habitats can be combined with rigid outer shells for added protection and durability.
Environmental and Health Challenges
Radiation Protection
Radiation Levels on Mars
Radiation protection is a critical concern for Mars missions due to the elevated levels of cosmic and solar radiation present on the planet. Unlike Earth, which has a robust magnetic field and thick atmosphere to shield against radiation, Mars has a much thinner atmosphere and lacks a global magnetic field. As a result, surface radiation levels on Mars are significantly higher than on Earth. Measurements from Mars missions have shown that the surface radiation on Mars can be about 100 times greater than the average levels on Earth, posing a serious health risk to humans.
Cosmic rays and solar particle events contribute to this high radiation environment. Cosmic rays are high-energy particles originating from outside the solar system, while solar particle events are bursts of high-energy particles from the Sun. Both types of radiation can penetrate spacecraft and habitat walls, potentially damaging human tissues and increasing the risk of cancer and other health issues.
Shielding Techniques and Technologies
To mitigate radiation exposure, several shielding techniques and technologies are employed in spacecraft and habitats. One approach is the use of thick, durable materials that can absorb or deflect radiation. For spacecraft, this includes incorporating layers of specialized materials, such as polyethylene or boron-infused composites, which are effective at blocking radiation.
On Mars, habitats may use regolith as a radiation shield. By constructing habitats with thick layers of Martian soil or utilizing inflatable structures covered with regolith, the habitat can provide substantial protection from radiation. Additionally, incorporating water tanks or other dense materials within the habitat’s walls can further enhance shielding.
Advanced technologies, such as active radiation shielding, are also being explored. These systems generate magnetic or electric fields around the habitat to deflect charged particles, mimicking the protective effects of Earth’s magnetic field. While still in the experimental stage, such technologies hold promise for improving radiation protection in future Mars missions.
Life Support Systems
Oxygen Production and Recycling
Life support systems are essential for maintaining a habitable environment on Mars, particularly in terms of oxygen production and recycling. On Mars, the atmosphere is composed of 95% carbon dioxide, making it unsuitable for human respiration. Therefore, a reliable system for producing oxygen is crucial. One method involves the use of chemical processes or electrolysis to convert carbon dioxide into oxygen. The Mars Oxygen In-Situ Resource Utilization Experiment (MOXIE) is an example of this technology, designed to extract oxygen from Martian carbon dioxide.
In addition to production, recycling systems are necessary to efficiently manage and reuse oxygen. Advanced systems are used to scrub carbon dioxide from the air and recycle it into oxygen and other useful gases. This closed-loop system minimizes the need for resupply from Earth and helps maintain a stable atmospheric composition within the habitat.
Water Purification and Management
Water is another critical resource that must be carefully managed in Mars habitats. Due to the limited availability of liquid water on Mars, efficient purification and recycling systems are essential. Water can be sourced from Martian ice or extracted from the atmosphere. Once obtained, it undergoes a series of purification processes to ensure it is safe for consumption.
Advanced filtration systems remove contaminants and impurities from the water. These systems include multi-stage filters and chemical treatments to ensure high water quality. Additionally, water recycling technologies reclaim moisture from the air, urine, and wastewater, converting it back into clean water. This closed-loop system is vital for sustainability, reducing the reliance on external water supplies and ensuring a consistent supply for the crew.
Psychological and Social Well-being
Mental Health Challenges in Isolation
The psychological and social well-being of crew members on Mars is a significant concern due to the challenges of isolation and confinement. Extended missions to Mars involve long durations of isolation from Earth and limited social interaction, which can lead to mental health issues such as depression, anxiety, and stress. The confined living quarters and lack of privacy can exacerbate these challenges, making it crucial to address mental health proactively.
Crew members may experience feelings of loneliness, claustrophobia, and sensory deprivation. The lack of direct contact with family and friends, combined with the monotonous environment, can impact morale and mental health. Understanding and mitigating these effects are essential for maintaining crew performance and overall mission success.
Strategies for Maintaining Social Interactions
To support psychological well-being, strategies for maintaining social interactions and mental health are implemented. Regular communication with loved ones on Earth through video calls and messages can help alleviate feelings of isolation and maintain emotional connections. Crew members can also engage in recreational activities, such as hobbies and exercise, to reduce stress and improve mood.
Psychological support services, including access to mental health professionals and counseling, are provided to address mental health concerns. Crew members are trained in stress management techniques and conflict resolution to handle interpersonal issues that may arise during the mission. Additionally, maintaining a structured daily routine and providing opportunities for personal and team activities contribute to a positive and supportive living environment.
Medical Challenges and Solutions
Health Risks of Long-Term Space Travel
Long-term space travel poses several health risks, including the effects of microgravity, radiation exposure, and isolation. Microgravity can lead to muscle atrophy, bone density loss, and cardiovascular issues. Crew members must engage in regular exercise and physical therapy to mitigate these effects and maintain physical health.
Radiation exposure, as discussed earlier, increases the risk of cancer and other health problems. Effective radiation shielding and monitoring are essential to protect crew members from harmful radiation levels.
Psychological challenges, such as stress and anxiety, can also impact health. Addressing mental health proactively and providing support services are crucial for maintaining overall well-being during long-duration missions.
Medical Facilities and Telemedicine
To address medical challenges, Mars missions will be equipped with advanced medical facilities and telemedicine capabilities. Medical facilities onboard will include diagnostic equipment, treatment tools, and supplies for handling common medical issues and emergencies. Crew members will receive training in basic medical procedures and first aid to manage health issues that may arise.
Telemedicine allows crew members to consult with Earth-based medical professionals for guidance and support. Real-time communication with experts can help diagnose and treat health problems, ensuring that crew members receive appropriate medical care despite the distance from Earth. Advanced telemedicine systems will include video consultations, data transmission, and remote monitoring to provide comprehensive medical support during Mars missions.
Logistical and Operational Challenges
Transportation and Supply Chain Management
Logistics of Transporting Supplies
Efficient logistics are crucial for Mars missions, as transporting supplies to and from the Red Planet involves significant challenges. The vast distance between Earth and Mars necessitates careful planning and coordination to ensure that essential supplies, including food, water, equipment, and scientific instruments, arrive safely and on time. This involves selecting appropriate launch windows when Earth and Mars are optimally aligned to minimize travel time and fuel consumption.
Transporting supplies requires robust spacecraft capable of carrying large payloads while withstanding the harsh conditions of space travel. Cargo spacecraft must be designed with precision to handle the stresses of launch, transit, and landing, and to protect the contents from potential contamination and damage. Additionally, integrating efficient packaging and storage solutions is critical to maximize space and ensure the preservation of sensitive materials during transit.
Ensuring a Sustainable Supply Chain
Sustainability is a key focus in managing the supply chain for Mars missions. Reducing dependency on Earth-based resupply is essential for long-term mission success. One approach is to develop in-situ resource utilization (ISRU) technologies to produce essential supplies on Mars. This includes extracting water from Martian ice, generating oxygen and fuel from the atmosphere, and growing food in controlled environments.
Building a sustainable supply chain also involves establishing protocols for recycling and reusing materials. Implementing closed-loop systems for water and air, along with recycling waste products, helps to minimize the need for resupply from Earth and reduces environmental impact. Additionally, fostering partnerships with private companies and international space agencies can enhance resource sharing and logistics capabilities, contributing to a more resilient supply chain.
Energy Production and Storage
Solar Power and Alternative Energy Sources
Energy production is a critical aspect of Mars missions, given the need for a continuous and reliable power supply. Solar power is the primary energy source for most Mars missions due to its availability and established technology. Solar panels are used to convert sunlight into electricity, powering spacecraft systems, habitats, and scientific instruments. However, solar power on Mars is less efficient than on Earth due to the planet’s greater distance from the Sun and frequent dust storms that can reduce sunlight exposure.
Alternative energy sources are also explored to complement solar power. Nuclear power, such as radioisotope thermoelectric generators (RTGs), provides a consistent energy supply and is less affected by environmental conditions. RTGs have been used successfully in Mars missions and other space exploration missions, offering a reliable energy source for extended operations. Additionally, advanced energy production technologies, such as small modular reactors, are being studied for their potential to provide long-term, stable power on Mars.
Energy Storage Solutions
Effective energy storage is essential for managing power supply on Mars, especially during periods when solar energy is not available. Energy storage solutions, such as high-capacity batteries and advanced energy storage systems, play a crucial role in ensuring a continuous power supply. Lithium-ion batteries are commonly used due to their high energy density and efficiency, but research into next-generation batteries, such as solid-state or flow batteries, aims to improve performance and longevity.
Another approach is to use energy storage in the form of chemical energy. This includes technologies like hydrogen fuel cells, which store energy in hydrogen and release it through a chemical reaction to generate electricity. Hydrogen fuel cells offer the advantage of providing power on demand and producing only water as a byproduct, making them a clean and efficient energy storage solution.
Communication Systems
Maintaining Communication with Earth
Maintaining reliable communication with Earth is critical for Mars missions, ensuring that data, commands, and updates are exchanged efficiently. Communication systems must be designed to handle the vast distances and varying signal strengths between Mars and Earth. High-gain antennas and powerful transmitters are used to send and receive signals, while ground stations on Earth are equipped with large dish antennas to capture signals from Mars.
To ensure continuous communication, spacecraft and habitats on Mars are equipped with redundant communication systems that can switch between different frequencies and protocols. This redundancy helps to maintain connectivity even if one system experiences issues or interference. Additionally, communication relays may be established using orbiting satellites around Mars to facilitate better signal coverage and transmission reliability.
Overcoming Time Delay Challenges
Time delays in communication between Mars and Earth are an inherent challenge due to the vast distance. Signals can take between 4 to 24 minutes to travel one way, depending on the relative positions of the two planets. To overcome this delay, mission operations must be carefully planned, with systems designed to operate autonomously when real-time communication is not possible.
Data transmission protocols are optimized to handle delays, including buffering and pre-scheduled command execution. Automated systems and onboard intelligence play a crucial role in managing routine operations and responding to emergencies without immediate input from Earth. Mission control on Earth must also account for these delays when planning and executing commands, ensuring that operations are synchronized with the time lag.
Robotics and Automation
Role of Robots in Construction and Maintenance
Robots play a vital role in the construction and maintenance of Mars habitats and infrastructure. These machines can perform tasks that are challenging or hazardous for humans, such as building habitats, conducting repairs, and exploring the Martian surface. Robotic systems designed for construction can use materials like Martian regolith to build structures, reducing the need for transporting materials from Earth.
Maintenance robots are equipped with tools and sensors to inspect, repair, and service equipment and habitats. These robots can perform routine maintenance tasks, such as cleaning and replacing components, to ensure the functionality and longevity of critical systems. Their ability to operate autonomously or semi-autonomously allows them to address issues promptly and efficiently, minimizing downtime and ensuring that habitats remain operational.
Autonomous Systems for Daily Operations
Autonomous systems are essential for managing daily operations on Mars, where communication delays and the need for continuous activity require systems that can operate independently. These systems include autonomous rovers, drones, and habitat management systems that perform tasks without constant human intervention.
Autonomous rovers are used for exploration, surveying, and resource extraction, equipped with advanced sensors and AI to navigate the Martian terrain and carry out scientific experiments. Drones and aerial robots can assist in surveying large areas, monitoring environmental conditions, and performing inspections.
Scientific Opportunities
Mars Geological Studies
Understanding Mars’ Geology
Mars geological studies aim to unravel the planet’s geological history and surface composition, offering insights into its formation and evolution. By analyzing Martian rocks, soil, and surface features, scientists can better understand the planet’s geological processes, including volcanism, erosion, and sedimentation. Key geological features on Mars, such as the massive volcano Olympus Mons and the vast canyon Valles Marineris, provide valuable information about the planet’s volcanic and tectonic activity.
Recent missions, such as NASA’s Mars rovers and orbiters, have conducted detailed analyses of Martian geology. Instruments on these missions have detected a variety of rock types, including sedimentary, igneous, and metamorphic, suggesting a complex history of geological activity. Studies of Martian minerals, such as clays and sulfates, have revealed evidence of past water activity, which is crucial for understanding Mars’ climatic history and potential habitability.
Potential for Resource Extraction
Mars’ geological studies also focus on identifying and assessing potential resources that could support future human missions and colonization. The presence of water ice, particularly at the polar regions and in the subsurface, is of great interest. Water is a critical resource for life support, agriculture, and the production of fuel through electrolysis.
In addition to water, scientists are investigating the presence of valuable minerals and metals on Mars. Elements such as iron, nickel, and platinum-group metals are considered important for supporting human infrastructure and technology. Identifying and extracting these resources could reduce the need for transporting materials from Earth, making Mars colonization more feasible and sustainable.
Search for Extraterrestrial Life
Current Evidence and Theories
The search for extraterrestrial life on Mars involves examining various lines of evidence and exploring theories about the planet’s potential to support life. Current evidence includes the detection of organic molecules, which are the building blocks of life, and the presence of seasonal methane fluctuations in the Martian atmosphere. Methane, a gas often associated with biological activity on Earth, has sparked interest in the possibility of microbial life on Mars.
Theories about Martian life include the idea that microbial organisms might have once existed in liquid water environments, such as ancient lakes or underground aquifers. The discovery of ancient riverbeds and lake deposits on Mars supports the hypothesis that the planet may have had habitable conditions in the past. Additionally, scientists consider the potential for life to exist in subsurface environments, where water might remain liquid due to geothermal heat.
Missions Aimed at Discovering Life
Several missions have been designed to search for signs of life on Mars, employing a variety of scientific techniques and instruments. NASA’s Perseverance rover, which landed on Mars in February 2021, is equipped with advanced tools to search for biosignatures and collect samples from the Martian surface. These samples are intended to be returned to Earth by future missions for more detailed analysis.
The European Space Agency’s ExoMars rover, set to launch in the coming years, will also contribute to the search for life by drilling into the Martian soil and analyzing samples for organic compounds and potential biosignatures. In addition, upcoming missions aim to explore the icy moons of Mars, such as Phobos and Deimos, for clues about the planet’s potential to support life.
Climate and Atmospheric Research
Studying Mars’ Climate and Weather Patterns
Understanding Mars’ climate and weather patterns is essential for planning future missions and assessing the feasibility of long-term colonization. Mars has a thin atmosphere composed mainly of carbon dioxide, with trace amounts of nitrogen and argon. This atmosphere contributes to extreme temperature variations, ranging from frigid lows near the poles to relatively warmer temperatures at the equator.
Mars experiences weather phenomena such as dust storms, which can cover the entire planet and last for weeks or months. These storms affect temperature, atmospheric pressure, and solar radiation, impacting both scientific observations and mission operations. Studying these weather patterns involves using data from orbiters and landers equipped with meteorological instruments to monitor atmospheric conditions and seasonal changes.
Implications for Future Colonization
The knowledge gained from studying Mars’ climate and weather patterns has significant implications for future colonization efforts. Understanding temperature fluctuations, radiation levels, and atmospheric conditions helps in designing habitats and life support systems that can withstand the planet’s extreme environment.
Effective climate modeling can aid in predicting weather events and planning mission activities, ensuring that habitats and equipment are prepared for conditions such as dust storms or temperature extremes. Additionally, insights into Martian climate patterns are crucial for developing strategies for resource management, including water extraction and energy production, which are vital for sustaining human life on Mars.
Political and Ethical Considerations
International Collaboration and Competition
Partnerships with Other Space Agencies
International collaboration is crucial for advancing Mars exploration and colonization efforts. Partnerships between space agencies can pool resources, share expertise, and reduce the financial burden of ambitious space missions. Notable examples of such collaboration include joint missions and research initiatives between NASA, the European Space Agency (ESA), and other national space organizations. These partnerships enable the integration of diverse technologies and scientific approaches, fostering innovation and enhancing mission capabilities.
Collaborative efforts often involve sharing data, research findings, and technological advancements. For instance, NASA’s Mars rovers have benefited from contributions by international teams that provide specialized instruments and analytical expertise. Additionally, the International Space Station (ISS) serves as a model for international cooperation in space, demonstrating the potential for joint efforts in developing and testing technologies that could be applied to Mars missions.
Collaborative projects also extend to the development of infrastructure and technology needed for Mars colonization. Working together on areas such as life support systems, propulsion technology, and habitat design allows space agencies to leverage each other’s strengths and accelerate progress. Joint missions and research agreements help establish a foundation for future Mars exploration and ensure that multiple nations can contribute to and benefit from the scientific and technological advancements made.
Competitive Landscape of Mars Exploration
The competitive landscape of Mars exploration is defined by the race among various space agencies and private companies to achieve significant milestones in Martian exploration. Nations like the United States, China, and Russia are actively pursuing their own Mars missions, each with unique objectives and strategies. China’s space agency, CNSA, has made notable strides with its Tianwen missions, aiming to establish a strong presence in Martian exploration.
Private companies, such as SpaceX, are also driving competition with ambitious CNSA Plans for Mars Colonization. SpaceX’s Starship, designed for interplanetary travel, represents a significant leap in spacecraft technology and mission capability. The competition among these entities fosters innovation, as each strives to achieve scientific breakthroughs and technological advancements that set them apart.
The competitive environment also stimulates investment in Mars research and technology development, pushing the boundaries of what is possible. While competition can lead to accelerated progress, it also underscores the need for coordination and collaboration to address common challenges and maximize the benefits of exploration. As various stakeholders vie for leadership in Mars exploration, the interplay between competition and collaboration will shape the future of interplanetary exploration.
Legal and Ethical Issues
Space Law and CNSA Plans for Mars Colonization
Space law plays a critical role in governing activities related to Mars colonization and exploration. International treaties, such as the Outer Space Treaty (1967) and the Moon Agreement (1984), provide a legal framework for space activities, emphasizing that outer space, including Mars, is the province of all humankind and cannot be claimed by any single nation. These treaties establish principles for the use of space resources, the protection of space environments, and the prevention of conflicts in space.
As Mars colonization progresses, new legal issues arise concerning the use of Martian resources, property rights, and governance structures. The question of who has the authority to claim or exploit Martian resources remains unresolved, as existing treaties do not fully address the complexities of resource utilization on other planetary bodies. Legal frameworks may need to evolve to address issues such as ownership rights, commercial activities, and environmental protection.
Developing clear and equitable space laws will be essential for managing international cooperation, preventing disputes, and ensuring the responsible use of Martian resources. Collaboration among space-faring nations and stakeholders will be necessary to establish comprehensive regulations that balance exploration, scientific research, and commercial interests.
Ethical Considerations of Human Presence on Mars
The ethical considerations of human presence on Mars involve several important aspects, including the impact on potential Martian life, the welfare of astronauts, and the long-term effects on the Martian environment. One key ethical concern is the possibility of contaminating Mars with Earth-based microbes, which could interfere with the search for indigenous life forms and disrupt any existing ecosystems. Planetary protection protocols are in place to minimize this risk, but ethical deliberations continue about the potential consequences of human activity on Mars.
The welfare of astronauts is another critical ethical consideration. Prolonged isolation, exposure to radiation, and psychological stress pose significant risks to the health and well-being of crew members. Ensuring adequate support systems, medical care, and mental health resources is essential to address these concerns and uphold the ethical responsibility of protecting the individuals undertaking these missions.
Finally, the long-term effects of colonization on the Martian environment must be considered. Ethical questions arise about the extent to which humanity should alter or terraform Mars and the potential consequences for the planet’s natural state. Balancing the pursuit of scientific and exploratory goals with the responsibility to preserve the Martian environment will be a key challenge in addressing the ethical dimensions of Mars colonization.
Economic Opportunities
Commercial Ventures and Private Sector Involvement
Role of Private Companies in Mars Missions
The role of private companies in Mars missions has become increasingly prominent, driven by advancements in technology and a growing interest in space exploration. Companies like SpaceX, Blue Origin, and others are spearheading ambitious plans for Mars colonization, leveraging their expertise in spacecraft design, propulsion technology, and mission planning. SpaceX, in particular, is at the forefront with its Starship spacecraft, designed for interplanetary travel and capable of carrying large payloads to Mars.
Private companies contribute to Mars missions through various means, including developing and manufacturing spacecraft, providing launch services, and investing in research and development. Their involvement accelerates technological advancements and introduces new approaches to space exploration. For example, SpaceX’s reusable rocket technology has significantly reduced the cost of access to space, making ambitious Mars missions more economically feasible.
Moreover, private sector participation fosters competition, which drives innovation and efficiency. By collaborating with governmental space agencies and other commercial entities, private companies help advance the overall capabilities of Mars exploration. Their contributions also include developing supporting technologies such as life support systems, habitat construction techniques, and in-situ resource utilization technologies.
Potential for Economic Growth and Innovation
The involvement of private companies in Mars missions holds significant potential for economic growth and innovation. As companies invest in developing new technologies and infrastructure for Mars exploration, they create opportunities for advancements in various fields, including materials science, robotics, artificial intelligence, and sustainable energy.
The commercial space sector’s growth generates new business opportunities and jobs, contributing to the expansion of the aerospace industry. Innovations developed for Mars missions can have broader applications on Earth, such as advancements in environmental technology, telecommunications, and manufacturing processes. For instance, technologies designed for life support and resource extraction on Mars could lead to improvements in resource management and sustainability on Earth.
Additionally, the commercialization of space travel and exploration opens new markets and revenue streams. Space tourism, asteroid mining, and lunar base development are potential areas for economic activity, driven by the advancements made during Mars missions. As the private sector continues to push the boundaries of space exploration, it fosters a dynamic and evolving space economy with far-reaching implications for both technological progress and economic growth.
Resource Utilization and Economic Benefits
Extracting and Utilizing Mars’ Resources
The extraction and utilization of Mars’ resources are central to the feasibility and sustainability of long-term human missions and colonization. Mars holds potential resources such as water ice, minerals, and gases that are essential for supporting human life and infrastructure. Water ice, found at the polar regions and in subsurface deposits, can be converted into drinking water, oxygen, and rocket fuel through electrolysis, reducing the need to transport these resources from Earth.
Mars’ regolith, or surface soil, contains valuable minerals and elements that could be used for construction and manufacturing. For example, iron, silicon, and aluminum found in Martian soil can be utilized to build habitats and equipment. Additionally, technologies for resource extraction and processing on Mars, such as mining and refining, are crucial for establishing a self-sufficient presence on the planet.
Efficient resource utilization involves developing technologies and systems that can operate in the harsh Martian environment. This includes designing equipment for resource extraction, processing, and storage, as well as establishing protocols for the sustainable management of Martian resources. By harnessing these resources, missions can reduce their reliance on Earth and achieve greater operational independence.
Economic Impact of Mars Colonization
The economic impact of Mars colonization extends beyond the initial investment in missions and infrastructure. Successful colonization efforts can drive economic growth through the development of new industries and technologies. The establishment of a permanent human presence on Mars creates opportunities for research and development, leading to innovations in science, engineering, and resource management.
Mars colonization also opens up new markets for commercial ventures. Space tourism, for instance, could become a viable industry as technology advances and costs decrease. The potential for mining and resource extraction on Mars may provide valuable materials for use on Earth and in space, contributing to economic growth.
Furthermore, the development of technologies for Mars colonization can have broader applications, impacting various sectors such as telecommunications, environmental management, and transportation. The advancements made in space exploration and resource utilization may lead to improvements in technology and infrastructure on Earth, driving economic benefits across multiple industries.
Conclusion
Recap of Key Points Discussed
CNSA Plans for Mars Colonization efforts represent a monumental undertaking in space exploration, characterized by both significant challenges and promising opportunities. The vision for Mars colonization includes long-term goals such as establishing a sustainable human presence, utilizing Martian resources, and advancing scientific knowledge. Key phases of this endeavor involve rigorous planning, technological development, and international collaboration.
Spacecraft design and development are central to Mars missions, with innovations in technology driving progress. Key considerations include propulsion systems, fuel efficiency, and habitat construction. Addressing these challenges involves overcoming technical obstacles related to radiation protection, life support systems, and the psychological well-being of astronauts.
The search for extraterrestrial life, climate and atmospheric research, and resource utilization are crucial areas of focus. Understanding Mars’ geology and climate helps inform mission planning and habitat design. Efforts to discover life and study Martian resources offer both scientific insights and practical benefits for future colonization.
International collaboration and competition shape the landscape of Mars exploration, with partnerships enhancing capabilities while competition drives innovation. Legal and ethical issues, including space law and the implications of human presence on Mars, are integral to ensuring responsible and sustainable exploration.
Private sector involvement and commercial ventures add a dynamic element to Mars missions, with companies contributing technology, investment, and innovation. The economic impact of Mars colonization includes potential growth in new industries and advancements that benefit both space exploration and terrestrial applications.
Outlook for CNSA’s Mars Colonization Efforts
CNSA’s Mars colonization efforts are poised to make significant strides in the coming years. The agency’s commitment to advancing space exploration technology and fostering international partnerships positions it as a key player in the global effort to explore and settle Mars. Continued investment in research, technology development, and mission planning will be critical to overcoming the technical and logistical challenges associated with Mars missions.
The outlook for CNSA’s Mars colonization efforts includes both ambitious milestones and the potential for groundbreaking discoveries. As the agency progresses through its planned missions, including landers, rovers, and potential crewed missions, it will contribute valuable data and experience to the broader scientific community.
The involvement of private companies and international collaboration will likely play a significant role in shaping the future of Mars colonization. By leveraging advancements in technology and fostering innovation, CNSA and its partners can address the complex challenges of Mars exploration and harness the opportunities for scientific discovery and economic growth.
FAQs
Common Questions about CNSA’s Mars Colonization Plans
What are the primary goals of CNSA’s Mars missions?
CNSA’s Mars missions are driven by several primary goals aimed at advancing space exploration and establishing a presence on the Red Planet. The foremost objective is to conduct thorough scientific research to better understand Mars’ geology, climate, and potential for life. This involves deploying sophisticated rovers and landers equipped with advanced instruments to analyze Martian soil, rocks, and atmospheric conditions.
Another key goal is to develop and test technologies necessary for future human missions and long-term colonization. This includes innovations in spacecraft design, life support systems, habitat construction, and in-situ resource utilization. By testing these technologies in the harsh Martian environment, CNSA aims to overcome the significant challenges of sustaining human life on Mars.
CNSA also seeks to establish a foundational presence on Mars by sending robotic missions that can pave the way for future crewed missions. This includes evaluating potential landing sites, conducting preliminary resource assessments, and developing infrastructure that will support human exploration and settlement. Long-term, CNSA envisions a sustainable human presence on Mars, contributing to the broader goal of interplanetary colonization.
How does CNSA plan to address the health challenges of Mars colonization?
Addressing the health challenges of Mars colonization is a critical aspect of CNSA’s mission planning. The agency recognizes that astronauts will face a range of health issues due to the Martian environment, including radiation exposure, psychological stress, and the effects of prolonged isolation.
To mitigate radiation risks, CNSA is investing in advanced shielding technologies and habitat designs that can protect astronauts from harmful cosmic and solar radiation. This includes designing habitats with radiation-resistant materials and exploring methods for creating protective magnetic fields.
Health and psychological well-being are also priorities for CNSA. The agency plans to implement robust life support systems that provide reliable oxygen production, water purification, and waste management. Additionally, strategies for mental health support will be integral, including regular communication with Earth, recreational activities, and psychological counseling to help astronauts cope with isolation and confinement.
Medical facilities and telemedicine are being developed to address potential health issues. This involves equipping missions with diagnostic tools and telemedicine capabilities that allow for remote consultations with medical experts on Earth. Ensuring that astronauts have access to medical care and emergency response capabilities is crucial for maintaining their health during extended missions.
What role will international cooperation play in CNSA’s Mars missions?
International cooperation is expected to play a significant role in CNSA’s Mars missions, enhancing the overall effectiveness and success of the exploration effort. Collaborative partnerships with other space agencies, such as NASA, ESA, and Roscosmos, allow CNSA to leverage a wide range of expertise, technologies, and resources.
Joint missions and research initiatives enable the sharing of data, technology, and best practices. This collaboration can lead to more efficient use of resources, reduce duplication of effort, and facilitate the integration of diverse scientific and technical approaches. For example, international partners may contribute specialized instruments or provide valuable insights into mission planning and execution.
International cooperation also helps in addressing the complex challenges of Mars exploration, such as the development of life support systems, habitat construction, and in-situ resource utilization. By working together, space agencies can pool their knowledge and experience to overcome technical obstacles and accelerate the progress of Mars missions.