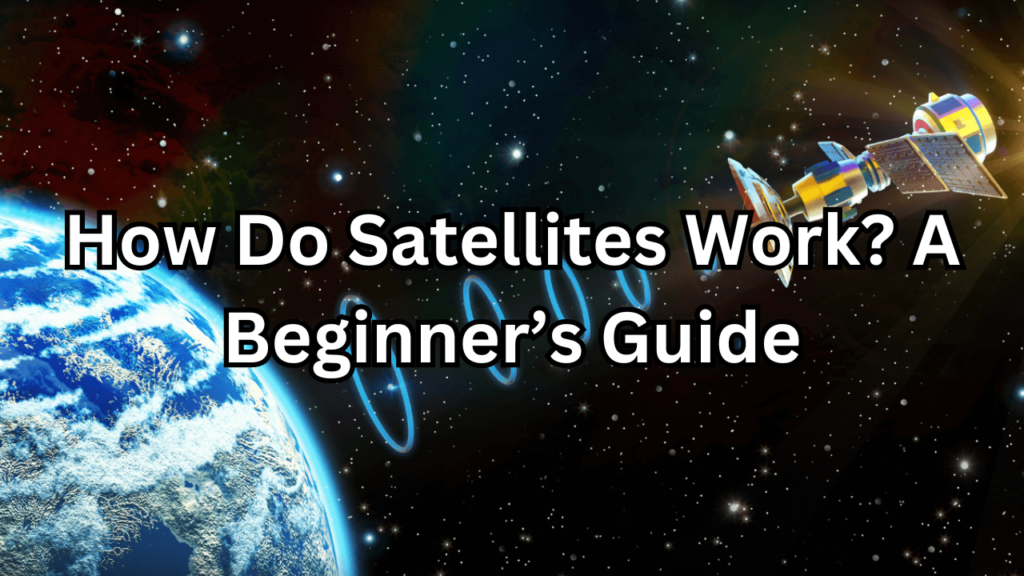
Table of Contents
Introduction
How Do Satellites Work, satellites play a crucial role in our modern world, serving as the backbone for a myriad of technologies and services we rely on daily. From enabling global communication networks to providing critical weather forecasts, these marvels of engineering have revolutionized the way we live, work, and interact with our environment. This beginner’s guide aims to demystify the complex world of satellites, offering a comprehensive overview of how they work, their history, and their indispensable role in contemporary society. By the end of this guide, readers will have a solid understanding of the fundamental principles behind satellite technology and its far-reaching impacts.
What is a Satellite?
A satellite is an artificial object intentionally placed into orbit around the Earth or another celestial body. These objects are designed to perform a variety of functions, from communications and navigation to Earth observation and scientific research. Satellites come in different shapes and sizes, each tailored to its specific mission requirements. They are equipped with an array of sophisticated instruments and systems, including power supplies, communication modules, sensors, and propulsion mechanisms, which enable them to operate autonomously in the harsh environment of space.
Brief History of Satellites
The journey of satellites began with the launch of Sputnik 1 by the Soviet Union on October 4, 1957. This historic event marked the dawn of the space age, demonstrating that it was possible to send objects into orbit around the Earth. Sputnik 1, a simple metal sphere with four external radio antennas, transmitted radio pulses back to Earth, capturing the imagination of the world. Following this, a series of advancements rapidly unfolded. The United States launched Explorer 1 in 1958, which discovered the Van Allen radiation belts. In the following decades, satellites evolved from simple transmitters to complex machines capable of imaging, weather forecasting, and global communications, profoundly shaping technological progress and international relations.
Importance of Satellites in Modern Society
Satellites have become indispensable tools in modern society, underpinning numerous aspects of our daily lives and global infrastructure. They facilitate instant communication across continents, enabling everything from international phone calls to live television broadcasts. Navigation satellites, like those in the Global Positioning System (GPS), provide precise location data that powers everything from smartphone maps to autonomous vehicles. In meteorology, weather satellites offer critical data that helps predict and monitor weather patterns, aiding in disaster preparedness and response. Additionally, Earth observation satellites monitor environmental changes, track natural resources, and support scientific research. The military also relies heavily on satellites for reconnaissance, communication, and navigation. In essence, satellites are integral to the functioning and advancement of modern civilization.
Basics of How Do Satellites Work
Definition and Overview
Satellites are artificial objects intentionally placed into orbit around Earth or other celestial bodies for various purposes. They are engineered to perform a multitude of tasks, such as communication, navigation, weather monitoring, and scientific research. Satellites operate by receiving and transmitting data, often in the form of radio signals, to and from ground stations. Their unique vantage point in space allows them to cover vast areas of the Earth’s surface, providing essential services and information that would be difficult or impossible to obtain from the ground.
Types of Satellites
Satellites can be categorized based on their function, orbit, and size. The primary types of satellites include communication satellites, weather satellites, navigation satellites, scientific satellites, and military satellites. Each type serves a distinct purpose and is equipped with specialized instruments and technologies to fulfill its mission. Understanding these different types helps us appreciate the diverse applications and contributions of satellite technology in various fields.
Communication Satellites
Communication satellites are designed to relay and amplify radio telecommunications signals between multiple locations on Earth. They are crucial for global broadcasting, internet services, telephone communications, and satellite television. These satellites typically orbit in the geostationary orbit, about 35,786 kilometers above the equator, where they remain fixed relative to a point on the Earth’s surface. This allows them to provide continuous coverage to specific areas, making them ideal for broadcasting and data transmission purposes.
Weather Satellites
Weather satellites monitor and forecast weather conditions by capturing images and data of the Earth’s atmosphere. They are equipped with instruments that measure cloud cover, surface temperatures, atmospheric water vapor, and other meteorological parameters. There are two main types of weather satellites: geostationary satellites, which provide continuous observation of the same area, and polar-orbiting satellites, which offer more detailed data by scanning the entire globe. The information collected by weather satellites is vital for predicting severe weather events, climate research, and environmental monitoring.
Navigation Satellites
Navigation satellites provide positioning, navigation, and timing (PNT) services, enabling users to determine their precise location anywhere on Earth. The Global Positioning System (GPS) is the most well-known example, consisting of a constellation of satellites that broadcast time-stamped signals. By calculating the time it takes for these signals to reach a receiver, the receiver can determine its exact position. Navigation satellites are essential for a wide range of applications, including aviation, maritime navigation, land transportation, and personal navigation devices.
Scientific Satellites
Scientific satellites are used for research and exploration, studying various phenomena in space and on Earth. These satellites carry instruments to observe celestial objects, measure cosmic radiation, and conduct experiments in microgravity. They contribute to our understanding of the universe, solar system, and Earth’s natural processes. Notable examples include the Hubble Space Telescope, which has provided unprecedented images of distant galaxies, and the Earth Observing System (EOS) satellites, which monitor Earth’s climate and environmental changes.
Military Satellites
Military satellites support defense and security operations, offering capabilities such as reconnaissance, surveillance, communications, navigation, and missile detection. These satellites enable military forces to gather intelligence, monitor potential threats, coordinate operations, and enhance situational awareness. They operate in various orbits depending on their mission, with some providing real-time imagery and others ensuring secure and reliable communication channels for military personnel.
Components of a Satellite
Satellites are complex machines composed of various subsystems that work together to achieve their mission objectives. The main components of a satellite include the power system, communication system, propulsion system, and sensors and instruments. Each subsystem plays a critical role in ensuring the satellite’s functionality, reliability, and longevity in space.
Power System
The power system provides the energy required for the satellite’s operations. Most satellites use solar panels to convert sunlight into electrical power, which is then stored in batteries for use when the satellite is in the Earth’s shadow. The power system must be designed to generate sufficient energy to support all onboard systems and instruments, ensuring continuous operation throughout the satellite’s mission life.
Communication System
The communication system enables the satellite to send and receive data to and from ground stations. This system includes antennas, transmitters, receivers, and modems. It is responsible for relaying information collected by the satellite’s sensors back to Earth, as well as receiving commands from mission control. Effective communication systems are crucial for maintaining contact with the satellite and ensuring its operations can be monitored and controlled from the ground.
Propulsion System
The propulsion system is used to adjust the satellite’s position and maintain its orbit. It typically includes thrusters and fuel tanks that provide the necessary force to perform maneuvers such as orbit insertion, station-keeping, and deorbiting. The propulsion system ensures the satellite remains in its designated orbit and can make any necessary adjustments to avoid collisions or optimize its operational efficiency.
Sensors and Instruments
Sensors and instruments are the payload of the satellite, designed to perform the specific tasks for which the satellite was launched. These may include cameras for imaging, radiometers for measuring radiation, spectrometers for analyzing chemical compositions, and various other scientific instruments. The type and configuration of sensors and instruments depend on the satellite’s mission, whether it is for communication, Earth observation, scientific research, or military applications.
How Satellites are Launched
Launch Vehicles
Launch vehicles, also known as rockets, are engineered to transport satellites and other payloads from Earth into space. They come in various sizes and configurations, tailored to different types of missions and payloads. The primary function of a launch vehicle is to provide the necessary thrust to overcome Earth’s gravity and place the satellite into its designated orbit. Launch vehicles consist of multiple stages, each with its own engines and fuel. As the rocket ascends, stages are sequentially jettisoned once their fuel is expended, reducing weight and allowing the remaining stages to accelerate the payload further. Prominent examples of launch vehicles include the Falcon 9 by SpaceX, the Ariane 5 by Arianespace, and the Atlas V by United Launch Alliance.
Launch Sites
Launch sites, or spaceports, are specially equipped facilities designed for the preparation, assembly, and launching of rockets. These sites are strategically located to optimize the efficiency and safety of launches. Factors influencing the location of a launch site include proximity to the equator, which provides a rotational boost, and the availability of large, uninhabited areas for safety in case of launch failures. Notable launch sites around the world include the Kennedy Space Center in the United States, Baikonur Cosmodrome in Kazakhstan, Guiana Space Centre in French Guiana, and Vostochny Cosmodrome in Russia. Each launch site features complex infrastructure, including launch pads, control centers, and vehicle assembly buildings.
The Launch Process
The launch process encompasses all activities from pre-launch preparation to the final deployment of the satellite in its designated orbit. This process involves meticulous planning, coordination, and execution to ensure the success of the mission. It includes several critical phases: pre-launch preparation, lift-off and ascent, and satellite deployment. Each phase is essential to overcoming the challenges of launching a satellite and placing it into the correct orbit.
Pre-Launch Preparation
Pre-launch preparation involves extensive planning and testing to ensure the readiness of the satellite, launch vehicle, and ground support systems. This phase includes the integration of the satellite with the launch vehicle, fueling of the rocket, and final system checks. Engineers conduct simulations and rehearsals to verify that all systems are functioning correctly and to identify and mitigate potential issues. Additionally, the launch window is carefully calculated to align with the satellite’s intended orbit and mission requirements. Final weather assessments are made to ensure conditions are suitable for a safe launch.
Lift-Off and Ascent
Lift-off and ascent are the most dynamic and critical phases of the launch process. At lift-off, the rocket’s engines ignite, generating immense thrust to propel the vehicle off the launch pad and into the sky. During ascent, the rocket must pass through several stages, each with its own set of challenges, including atmospheric drag and gravitational pull. As the rocket climbs higher, it sheds its stages to reduce weight and increase efficiency. The ascent phase requires precise control to maintain the correct trajectory and velocity needed to reach the designated orbit. This phase concludes when the rocket has reached the desired altitude and velocity, positioning it for the next phase of the mission.
Satellite Deployment
Satellite deployment is the final phase of the launch process, where the satellite is released from the launch vehicle and placed into its designated orbit. This phase involves the careful separation of the satellite from the rocket, ensuring that it is positioned correctly and begins its intended mission. Deployment mechanisms, such as springs or small thrusters, are used to gently push the satellite away from the rocket. Once deployed, the satellite activates its onboard systems, extends solar panels if necessary, and begins communication with ground control. This phase marks the transition from launch to operational status, where the satellite starts performing its intended functions, whether it be communication, observation, navigation, or scientific research.
Satellite Orbits How Do Satellites Work
Types of Orbits
Satellite Orbits are placed in various types of orbits, each serving specific purposes and offering unique advantages. The main types of orbits include Low Earth Orbit (LEO), Medium Earth Orbit (MEO), Geostationary Orbit (GEO), and Polar Orbit. The choice of orbit depends on the satellite’s mission, desired coverage area, and operational requirements. Understanding the characteristics and benefits of each orbit type is essential for optimizing satellite performance and mission success.
Low Earth Orbit (LEO)
Low Earth Orbit (LEO) is the region of space within 2,000 kilometers (1,243 miles) of the Earth’s surface. Satellites in LEO travel at high speeds, completing an orbit in approximately 90 to 120 minutes. This orbit is ideal for Earth observation, scientific research, and certain communication applications. The close proximity to Earth allows for high-resolution imaging and low-latency communication. However, satellites in LEO experience atmospheric drag, which can lead to gradual orbital decay and requires periodic adjustments to maintain their altitude.
Medium Earth Orbit (MEO)
Medium Earth Orbit (MEO) is situated between LEO and GEO, typically ranging from 2,000 to 35,786 kilometers (1,243 to 22,236 miles) above Earth. Satellites in MEO are commonly used for navigation systems, such as the Global Positioning System (GPS), GLONASS, and Galileo. These orbits provide a balance between coverage area and signal delay. Satellites in MEO have longer orbital periods compared to LEO, usually around 12 hours, allowing them to cover larger regions of the Earth’s surface while still maintaining relatively low latency in communication.
Geostationary Orbit (GEO)
Geostationary Orbit (GEO) is a circular orbit located approximately 35,786 kilometers (22,236 miles) above the equator. Satellites in GEO have an orbital period equal to Earth’s rotation period, allowing them to remain fixed above a specific point on the equator. This stationary position makes GEO ideal for communication, weather monitoring, and broadcasting services. GEO satellites provide continuous coverage to the same geographic area, making them essential for television broadcasts, weather forecasting, and global communication networks. However, the high altitude results in a longer signal travel time, leading to higher latency compared to LEO and MEO.
Polar Orbit
Polar Orbit is a type of low Earth orbit that passes over the Earth’s poles, allowing the satellite to observe nearly every part of the Earth’s surface over time. As the Earth rotates beneath the satellite, it provides comprehensive coverage of the entire planet. Polar orbits are commonly used for Earth observation, environmental monitoring, and reconnaissance missions. The advantage of polar orbits is their ability to capture data from all latitudes, making them ideal for global mapping and weather prediction. Satellites in polar orbit can capture high-resolution images and collect detailed data on a wide range of environmental parameters.
Choosing the Right Orbit for a Satellite
Selecting the appropriate orbit for a satellite is a critical decision that depends on the mission objectives, desired coverage area, and technical requirements. Factors to consider include the type of data to be collected, the geographic regions of interest, the need for real-time communication, and the operational lifespan of the satellite. For instance, Earth observation missions benefit from LEO due to high-resolution imaging capabilities, while global communication networks prefer GEO for continuous coverage. Navigation systems like GPS utilize MEO to balance coverage and signal delay. Polar orbits are chosen for missions requiring global data collection, such as climate monitoring and reconnaissance.
Orbital Mechanics
Orbital mechanics, or astrodynamics, is the study of the motions of artificial satellites and other space objects under the influence of gravitational forces. It involves understanding and predicting the trajectories, velocities, and positions of satellites in their orbits. Key concepts in orbital mechanics include Kepler’s laws of planetary motion, which describe the elliptical orbits of celestial bodies, and Newton’s law of gravitation, which governs the forces acting on satellites. Orbital mechanics is essential for planning satellite launches, performing orbital maneuvers, and maintaining the desired orbit throughout the satellite’s operational life. Understanding these principles ensures that satellites achieve and maintain their intended orbits, optimizing their performance and mission success.
How Satellites Stay in Orbit
Gravitational Forces
Gravitational forces play a fundamental role in keeping satellites in orbit around the Earth. According to Newton’s law of universal gravitation, every object in the universe attracts every other object with a force that is directly proportional to the product of their masses and inversely proportional to the square of the distance between their centers. For satellites, this force is primarily exerted by the Earth, pulling the satellite toward its center. This gravitational pull is counteracted by the satellite’s forward motion, creating a stable orbit where the satellite continuously falls towards the Earth but also moves forward, resulting in a curved path around the planet. The balance between gravitational forces and the satellite’s inertia keeps it in orbit.
Orbital Velocity
Orbital velocity is the speed at which a satellite must travel to maintain its orbit around the Earth. This velocity depends on the altitude of the orbit; the closer the satellite is to the Earth, the faster it needs to move to counteract the stronger gravitational pull. For instance, satellites in low Earth orbit (LEO) travel at speeds of approximately 7.8 kilometers per second (17,500 miles per hour), while those in geostationary orbit (GEO) move at about 3.1 kilometers per second (7,000 miles per hour). Achieving and maintaining the correct orbital velocity is crucial for ensuring the satellite remains in its designated orbit without drifting away or falling back to Earth. This velocity is calculated based on the principles of orbital mechanics and ensures a delicate balance between gravitational forces and the satellite’s inertia.
Station-Keeping and Adjustments
Station-keeping refers to the maneuvers a satellite performs to maintain its designated position and orbit. Over time, various factors such as gravitational perturbations from the moon and the sun, solar radiation pressure, and atmospheric drag (in lower orbits) can cause a satellite to drift from its intended orbit. To counteract these effects, satellites are equipped with propulsion systems that allow them to make small adjustments. These adjustments, known as thruster burns, correct the satellite’s position and velocity, ensuring it stays within its operational parameters. Regular station-keeping maneuvers are essential for satellites in geostationary orbit, which must remain fixed relative to a point on the Earth’s surface, and for those in low Earth orbits, which may experience more significant drag and perturbations. Effective station-keeping ensures the satellite continues to perform its mission reliably and efficiently throughout its operational life.
Communication with Satellites
Ground Stations
Ground stations are critical facilities responsible for communicating with satellites and managing their operations. These stations are equipped with large antennas and sophisticated technology to transmit commands to satellites and receive data from them. Ground stations can range from small tracking stations to large, fully-equipped centers capable of handling multiple satellites simultaneously. They play a vital role in monitoring satellite health, updating software, and processing the data sent back from space. Ground stations are strategically located around the world to ensure continuous contact with satellites as they orbit the Earth, providing essential support for satellite missions and ensuring the smooth operation of space-based systems.
Uplinks and Downlinks
Uplinks and downlinks are the communication processes between a satellite and a ground station. An uplink is the transmission of data or commands from the ground station to the satellite. This might include instructions to adjust the satellite’s orientation, perform specific tasks, or change its operational mode. A downlink is the transmission of data from the satellite back to the ground station. This data might include scientific measurements, images, or other information collected by the satellite’s instruments. The effectiveness of uplinks and downlinks depends on the quality of the ground station equipment, the satellite’s communication systems, and the atmospheric conditions between the satellite and ground station.
Frequency Bands
Frequency bands refer to specific ranges of electromagnetic frequencies used for satellite communication. Different frequency bands offer varying benefits in terms of data transmission rates, signal strength, and susceptibility to interference. The choice of frequency band is crucial for optimizing satellite performance and achieving reliable communication. Common frequency bands used in satellite communication include L-Band, S-Band, C-Band, Ku-Band, and Ka-Band.
L-Band
L-Band covers frequencies between 1 to 2 GHz and is often used for mobile satellite services, including GPS and satellite phones. This band provides good penetration through atmospheric conditions, such as rain and clouds, making it suitable for mobile and handheld devices. However, L-Band offers relatively lower data rates compared to higher frequency bands, limiting its use for high-bandwidth applications.
S-Band
S-Band operates between 2 to 4 GHz and is used for a variety of satellite communications, including weather satellites and some communication satellites. It provides better data rates than L-Band and offers a good balance between signal propagation and bandwidth. S-Band is also less affected by weather conditions compared to higher frequency bands, making it suitable for reliable communications in adverse weather.
C-Band
C-Band spans frequencies from 4 to 8 GHz and is widely used for television broadcasting, satellite communications, and weather radar systems. It offers a good compromise between bandwidth and signal attenuation. C-Band signals are less susceptible to rain fade, making them reliable for long-distance and high-availability communications. However, the C-Band spectrum is becoming increasingly congested due to its widespread use.
Ku-Band
Ku-Band covers frequencies from 12 to 18 GHz and is commonly used for satellite television broadcasting, broadband internet, and communications. It provides higher data rates compared to C-Band and allows for smaller, more efficient antennas. However, Ku-Band signals are more susceptible to rain fade and other atmospheric conditions, which can affect signal quality during adverse weather.
Ka-Band
Ka-Band operates between 26.5 to 40 GHz and offers high data rates and increased bandwidth, making it ideal for high-capacity communication systems, such as advanced satellite internet services and high-definition television. The Ka-Band allows for even smaller antennas and provides faster data transmission. However, Ka-Band signals are highly sensitive to rain and atmospheric attenuation, which can impact signal reliability during heavy weather conditions.
Signal Processing
Signal processing involves the techniques and technologies used to encode, decode, and analyze the data transmitted between satellites and ground stations. It encompasses various methods for filtering, amplifying, and demodulating signals to ensure they are accurately transmitted and received. Signal processing is crucial for maintaining the integrity of communication, enhancing data quality, and minimizing interference. Advanced signal processing techniques, such as error correction and modulation schemes, help to optimize data transmission, improve signal clarity, and ensure reliable communication over vast distances. This process is essential for effective satellite operations and the successful transmission of information from space to Earth.
Applications of Satellites
Communication
Communication satellites facilitate the transmission of data, voice, and video signals across vast distances. These satellites serve as relay stations, receiving signals from one location and transmitting them to another, often thousands of kilometers away. They enable global connectivity, supporting various applications such as television broadcasting, internet services, and telephony. By providing coverage in remote and underserved regions, communication satellites ensure that people around the world can access vital information, entertainment, and communication services. Their ability to deliver consistent and reliable connections is fundamental to modern communication infrastructure.
Television Broadcasting
Television broadcasting via satellites involves the transmission of TV signals from a satellite to television receivers on the ground. Communication satellites in geostationary orbit are commonly used for this purpose, as their fixed position relative to a specific point on Earth allows them to provide continuous coverage to the same geographic area. This setup enables broadcasters to reach audiences across entire continents or globally, delivering live broadcasts, entertainment, news, and other programming. The use of satellites for television broadcasting has revolutionized the media industry, providing diverse and high-quality content to millions of viewers around the world.
Internet and Data Services
Satellites play a crucial role in providing internet and data services, particularly in areas where terrestrial infrastructure is limited or unavailable. Satellite internet services use communication satellites to relay data between ground stations and user terminals. This technology enables high-speed internet access in remote regions, maritime environments, and during emergency situations. Data services provided by satellites include broadband internet, virtual private networks (VPNs), and cloud computing. As demand for connectivity continues to grow, advancements in satellite technology, such as high-throughput satellites (HTS) and low Earth orbit (LEO) constellations, are enhancing the speed, capacity, and reliability of satellite-based internet services.
Telephony
Satellite telephony involves using satellites to transmit voice signals for telephone communication. This technology allows for global voice communication, including in remote or isolated regions where traditional telephone infrastructure is absent. Satellite phones, which operate using communication satellites, offer a reliable means of communication in emergencies, expeditions, and locations with limited connectivity. By leveraging satellites, telephony services can provide coverage in areas where terrestrial networks are impractical or economically unfeasible, ensuring connectivity regardless of geographic challenges.
Navigation
Navigation satellites provide positioning, navigation, and timing (PNT) services that are essential for a wide range of applications, from personal GPS devices to advanced autonomous systems. These satellites transmit signals that allow receivers to calculate their precise location, speed, and time. The accuracy and reliability of navigation systems have transformed industries such as transportation, aviation, and logistics, providing real-time tracking and navigation capabilities that enhance safety and efficiency.
GPS
The Global Positioning System (GPS) is a satellite-based navigation system operated by the United States. It consists of a constellation of at least 24 satellites in medium Earth orbit, working together to provide accurate positioning, navigation, and timing information worldwide. GPS signals are transmitted from satellites to ground-based receivers, which use the time delay of the signals to calculate their precise location. GPS is widely used in various applications, including navigation for vehicles, aircraft, and ships, as well as in mobile phones, mapping services, and surveying.
GLONASS
GLONASS (Global Navigation Satellite System) is Russia’s satellite navigation system, serving as an alternative to the U.S. GPS. It consists of a constellation of satellites in medium Earth orbit, similar to GPS, and provides global positioning, navigation, and timing services. GLONASS signals are used for various applications, including navigation for vehicles, aviation, and military operations. The system offers global coverage and is particularly valuable in regions where GPS coverage may be limited or in situations where redundancy is required for enhanced reliability.
Galileo
Galileo is the European Union’s global satellite navigation system, designed to provide accurate and reliable positioning and timing information worldwide. Unlike GPS and GLONASS, Galileo is a civilian system, with a focus on providing high-precision services to users. The Galileo constellation will eventually consist of 30 satellites in medium Earth orbit, offering enhanced accuracy and reliability. Galileo is designed to be interoperable with GPS and GLONASS, improving navigation and positioning services globally. It supports a range of applications, including transportation, emergency response, and scientific research.
Weather Monitoring
Weather monitoring through satellites involves observing and analyzing atmospheric conditions to forecast weather patterns and track meteorological phenomena. Satellites equipped with sensors and imaging devices capture data on cloud cover, temperature, humidity, and atmospheric pressure. This data is crucial for predicting weather changes, issuing severe weather warnings, and understanding long-term climate trends. Weather satellites operate in various orbits, including geostationary and polar orbits, to provide continuous and global coverage, enabling meteorologists to make accurate weather forecasts and respond to natural events.
Meteorology
Meteorology is the scientific study of the atmosphere and its processes, focusing on weather and climate patterns. Satellites play a significant role in meteorology by providing real-time data on atmospheric conditions, weather systems, and climatic changes. This data aids meteorologists in analyzing weather patterns, forecasting short-term and long-term weather, and studying the impacts of climate change. Advanced satellite technologies, including infrared and microwave sensors, enhance the accuracy of weather predictions and contribute to a deeper understanding of atmospheric dynamics and their effects on global climate.
Climate Research
Climate research involves studying long-term atmospheric and environmental trends to understand climate change and its impacts. Satellites contribute valuable data for climate research by monitoring global temperature changes, sea level rise, ice cover, and greenhouse gas concentrations. This data is used to model climate systems, assess the effects of human activities on the environment, and develop strategies for mitigating climate change. Satellites provide a comprehensive view of the Earth’s climate, enabling researchers to track changes over time and inform policy decisions aimed at addressing climate-related challenges.
Earth Observation
Earth observation refers to the use of satellites to monitor and analyze the Earth’s surface and atmosphere. Satellites equipped with various sensors and imaging technologies collect data on land use, vegetation, water bodies, and urban development. This information supports applications such as environmental monitoring, resource management, and urban planning. Earth observation satellites operate in different orbits to provide detailed and comprehensive coverage, offering insights into natural and human-induced changes on the planet.
Environmental Monitoring
Environmental monitoring involves tracking and assessing the health of ecosystems, natural resources, and environmental conditions. Satellites provide critical data for environmental monitoring by measuring parameters such as air and water quality, deforestation, and pollution levels. This data helps in assessing the impact of human activities on the environment, detecting changes in natural habitats, and guiding conservation efforts. By providing timely and accurate information, satellites play a crucial role in protecting and managing the Earth’s environment.
Disaster Management
Disaster management utilizes satellite data to prepare for, respond to, and recover from natural and man-made disasters. Satellites offer real-time imagery and information on disasters such as hurricanes, earthquakes, floods, and wildfires. This data assists in assessing damage, coordinating emergency response efforts, and planning recovery strategies. Satellites also support disaster preparedness by providing early warnings and monitoring potential risks. Their ability to offer comprehensive coverage and timely updates is essential for effective disaster management and response.
Scientific Research
Scientific research involving satellites encompasses a wide range of disciplines, including Earth sciences, astronomy, and space exploration. Satellites provide valuable data for investigating various scientific phenomena, from studying Earth’s climate and natural resources to exploring outer space. Instruments aboard satellites enable researchers to conduct experiments, gather observations, and test hypotheses in areas that are challenging or impossible to access directly. Satellite-based scientific research contributes to advancements in knowledge and technology, driving innovation and discovery across multiple fields.
Space Exploration
Space exploration involves the study and exploration of outer space through the use of spacecraft, satellites, and other space technologies. Satellites play a crucial role in space exploration by providing data on cosmic phenomena, planetary bodies, and the space environment. They support missions aimed at understanding the origins of the universe, exploring other planets and moons, and studying cosmic events. Space exploration satellites enable scientists to investigate space conditions, conduct experiments in space, and advance our understanding of the universe and our place within it.
Astrophysics
Astrophysics is the branch of astronomy that focuses on understanding the physical properties and processes of celestial objects and phenomena. Satellites equipped with specialized instruments, such as telescopes and spectrometers, are essential for astrophysical research. They observe distant stars, galaxies, black holes, and other cosmic entities, providing data that helps scientists understand their composition, behavior, and evolution. Astrophysics research using satellites contributes to our knowledge of the fundamental laws of the universe, the formation of celestial bodies, and the nature of cosmic events.
Satellite Maintenance and End-of-Life
In-Orbit Servicing
In-orbit servicing refers to the maintenance, repair, and upgrade of satellites while they are in space. This capability is essential for extending the operational lifespan of satellites and ensuring their continued functionality. In-orbit servicing can involve a range of activities, including refueling, replacing components, and performing repairs on damaged systems. Advances in robotics, artificial intelligence, and space technologies have made it possible to carry out these tasks with increasing precision. This service helps to mitigate the costs and challenges associated with launching new satellites and allows for the enhancement of existing satellite missions. In-orbit servicing is a key component of future space operations, contributing to the sustainability and efficiency of satellite fleets.
Dealing with Space Debris
Space debris, or space junk, consists of defunct satellites, spent rocket stages, and fragments from collisions or disintegration of space objects. This debris poses significant risks to operational satellites and spacecraft, as even small fragments can cause substantial damage at high velocities. To manage and mitigate the threat of space debris, various strategies are employed, including debris tracking and monitoring systems, collision avoidance maneuvers, and the development of technologies for debris removal. Initiatives such as space debris mitigation guidelines and international cooperation aim to reduce the creation of new debris and address the existing clutter in Earth’s orbit. Effective management of space debris is crucial for ensuring the safety and sustainability of space operations.
Satellite Decommissioning
Satellite decommissioning is the process of safely ending a satellite’s operational life and removing it from active service. This process involves several steps to ensure that the satellite is removed from its operational orbit in a controlled manner and does not contribute to space debris. For satellites in low Earth orbit (LEO), decommissioning typically involves lowering the satellite’s orbit until it re-enters Earth’s atmosphere and burns up. In geostationary orbit (GEO), the satellite is moved to a “graveyard orbit” where it poses minimal risk to operational satellites. Proper decommissioning procedures are essential for maintaining space sustainability and preventing collisions or interference with other space assets.
Innovations in Satellite Technology
Miniaturization and CubeSats
Miniaturization in satellite technology has led to the development of small, cost-effective satellites known as CubeSats. CubeSats are typically built in standardized 10x10x10 cm units, known as “1U” cubes, and can be scaled to larger configurations by stacking multiple units. Their compact size and modular design make them ideal for a range of applications, including Earth observation, scientific research, and technology demonstration. The affordability and versatility of CubeSats allow for rapid prototyping and deployment, providing valuable opportunities for educational institutions, startups, and space agencies to experiment with new technologies and conduct space missions. Advances in miniaturization continue to expand the capabilities of CubeSats, making them a popular choice for both commercial and scientific missions.
Advances in Propulsion Systems
Advancements in propulsion systems are transforming satellite operations by enabling more efficient, precise, and sustainable space missions. Traditional chemical propulsion systems are being complemented by innovative technologies such as electric and ion propulsion. Electric propulsion systems, including Hall effect thrusters and ion thrusters, offer higher efficiency and greater specific impulse, allowing satellites to achieve higher velocities and extend their operational lifespans. Additionally, the development of advanced propulsion technologies, such as solar sails and hybrid systems, is enhancing the versatility and performance of satellites. These advancements contribute to improved maneuverability, extended mission durations, and the capability to undertake complex space missions with greater precision.
Improved Communication Technologies
Improved communication technologies are significantly enhancing satellite data transmission and connectivity. Advances in satellite communication include higher frequency bands, such as Ka-Band and Q/V-Band, which provide greater bandwidth and faster data rates. The development of high-throughput satellites (HTS) and low Earth orbit (LEO) constellations further boosts communication capabilities by increasing capacity and reducing latency. Innovations in beamforming, frequency reuse, and advanced modulation techniques also contribute to more efficient and reliable data transmission. These improvements enable better support for applications such as broadband internet, real-time communication, and global connectivity, meeting the growing demand for high-speed and reliable satellite services.
Future Trends
The future of satellite technology is shaped by several emerging trends and innovations. One key trend is the expansion of mega-constellations of small satellites, which aim to provide global broadband coverage and enhance connectivity in underserved regions. Advances in artificial intelligence and machine learning are also being integrated into satellite operations, enabling autonomous data processing, anomaly detection, and optimized mission planning. Additionally, the development of on-orbit manufacturing and in-orbit servicing technologies promises to revolutionize satellite maintenance and assembly. As the space industry continues to evolve, increased collaboration between public and private sectors, along with a focus on sustainability and space debris management, will play crucial roles in shaping the future of satellite missions and space exploration.
Challenges and Future of Satellites
Space Traffic Management
Space traffic management (STM) involves coordinating and regulating the movement of satellites and other space objects to ensure safe and efficient use of space. As the number of satellites and space missions increases, managing space traffic becomes increasingly complex. STM encompasses monitoring and tracking space objects, predicting potential collisions, and coordinating maneuvers to avoid collisions. Advanced tracking systems, such as radar and space-based sensors, provide data to help operators make informed decisions about satellite movements. Effective space traffic management is essential for preventing collisions, mitigating space debris, and maintaining the safety and sustainability of space operations.
International Regulations and Policies
International regulations and policies govern the use of outer space and are crucial for ensuring peaceful and orderly space activities. Key frameworks include the Outer Space Treaty (1967), which establishes the principles of non-appropriation of outer space, and the Convention on Registration of Objects Launched into Outer Space (1976), which mandates the registration of space objects with the United Nations. Additionally, guidelines for space debris mitigation and the guidelines established by the International Telecommunication Union (ITU) for frequency spectrum allocation are essential for managing space activities. These regulations and policies aim to promote cooperation, prevent conflicts, and address challenges related to space exploration and satellite operations.
Sustainability in Space
Sustainability in space focuses on ensuring that space activities are conducted in a manner that preserves the space environment for future generations. This includes addressing the challenges posed by space debris, minimizing the creation of new debris, and implementing measures to protect the space environment. Sustainable practices involve designing satellites with end-of-life disposal plans, adopting debris mitigation strategies, and encouraging international cooperation to develop and implement effective guidelines. The goal is to balance the benefits of space activities with the need to protect and maintain a safe and usable space environment for future missions and space exploration.
Upcoming Satellite Missions
Upcoming satellite missions represent the next wave of advancements in space technology and exploration. These missions encompass a diverse range of objectives, from scientific research and Earth observation to communications and space exploration. Notable upcoming missions include the deployment of mega-constellations for global broadband coverage, missions to study climate change and environmental changes, and exploration missions to the Moon, Mars, and beyond. Advances in satellite technology, such as miniaturization and improved propulsion systems, are enabling more ambitious and cost-effective missions. These future missions will play a crucial role in expanding our understanding of space, improving global connectivity, and addressing pressing challenges on Earth.
Conclusion
Recap of Key Points
How Do Satellites Work, satellites have become integral to modern life, influencing various sectors from communication and navigation to weather monitoring and scientific research. Key points about satellites include their diverse types and functions, such as communication satellites that facilitate global connectivity, weather satellites that provide crucial meteorological data, and navigation satellites that support systems like GPS and GLONASS. The advancement of satellite technology has led to miniaturization with CubeSats, innovations in propulsion systems, and improved communication technologies. Effective space traffic management, international regulations, and sustainability practices are essential to maintaining a safe and sustainable space environment. As we look to the future, emerging trends and upcoming missions promise to expand the capabilities and applications of satellites, shaping the way we interact with and understand our world.
The Future of Satellites and Their Impact on Society
The future of satellites is poised to bring transformative changes across various aspects of society. As technology advances, we can expect to see a proliferation of small satellites and mega-constellations that will enhance global connectivity, providing high-speed internet access to underserved and remote areas. Innovations in satellite technology, such as improved propulsion systems and on-orbit servicing, will increase the efficiency and longevity of space missions. The development of advanced data processing and artificial intelligence will enable more sophisticated applications in Earth observation, disaster management, and scientific research.
The impact of these advancements will be profound, offering new opportunities for economic growth, enhancing communication and information accessibility, and improving our ability to monitor and respond to environmental and humanitarian challenges. By addressing issues like space debris and promoting sustainability, the space industry will work towards preserving the space environment for future generations. Overall, the evolving capabilities of satellites will continue to drive progress, contribute to scientific discovery, and support a more connected and informed global society.
FAQs
How Long Do Satellites Typically Stay in Orbit?
The operational lifespan of satellites varies depending on their design, purpose, and orbit. In low Earth orbit (LEO), satellites generally stay in orbit for about 5 to 10 years before atmospheric drag or other factors cause them to re-enter the Earth’s atmosphere. Satellites in medium Earth orbit (MEO) and geostationary orbit (GEO) can remain operational for 10 to 15 years or longer due to their higher altitudes, which minimize atmospheric drag and environmental effects. However, their lifespans can be extended through advancements in technology and in-orbit servicing. The longevity of a satellite also depends on its ability to maintain its operational systems and withstand space conditions.
Can Satellites Collide with Each Other?
Yes, satellites can collide with each other, which is a significant concern in space traffic management. The increasing number of satellites and space debris raises the risk of collisions, which can create additional debris and exacerbate the problem. To mitigate this risk, space agencies and operators use tracking systems to monitor the positions of satellites and debris. In some cases, collision avoidance maneuvers are executed to prevent potential impacts. International cooperation and guidelines for debris mitigation are also in place to reduce the likelihood of collisions and maintain a safe operational environment in space.
How Are Satellites Powered?
Satellites are primarily powered by solar panels, which convert sunlight into electrical energy. These panels are equipped with photovoltaic cells that generate electricity, which is then used to power the satellite’s systems and instruments. Satellites also have onboard batteries that store energy generated by the solar panels, providing power during periods when the satellite is in the Earth’s shadow. Additionally, some satellites may use alternative power sources, such as radioisotope thermoelectric generators (RTGs) for deep-space missions, though these are less common. The power system design ensures that the satellite remains operational and can perform its functions throughout its mission life.
What Happens to Old or Non-Functional Satellites?
Old or non-functional satellites are managed based on their orbit and mission status. For satellites in low Earth orbit (LEO), they are typically deorbited by lowering their altitude until they re-enter the Earth’s atmosphere and burn up. In geostationary orbit (GEO), non-functional satellites are moved to a “graveyard orbit” away from operational satellites to minimize collision risks. If a satellite is still in a useful condition, it may be repurposed or decommissioned with proper procedures to prevent creating additional space debris. Ongoing efforts in space debris management and sustainability focus on improving these processes to reduce the environmental impact of defunct satellites.
How Do Satellites Affect Daily Life on Earth?
Satellites significantly impact daily life on Earth in numerous ways. Communication satellites provide essential services such as television broadcasting, internet access, and telephony, enabling global connectivity and access to information. Navigation satellites, such as those in the GPS and GLONASS systems, offer precise location and timing services that are crucial for transportation, logistics, and personal navigation. Weather satellites contribute to accurate weather forecasting, disaster monitoring, and climate research, enhancing safety and preparedness for natural events. Additionally, satellites play a role in environmental monitoring, scientific research, and space exploration, driving advancements in technology and improving our understanding of the planet and beyond. Overall, satellites support various aspects of modern life, enhancing convenience, safety, and global connectivity.