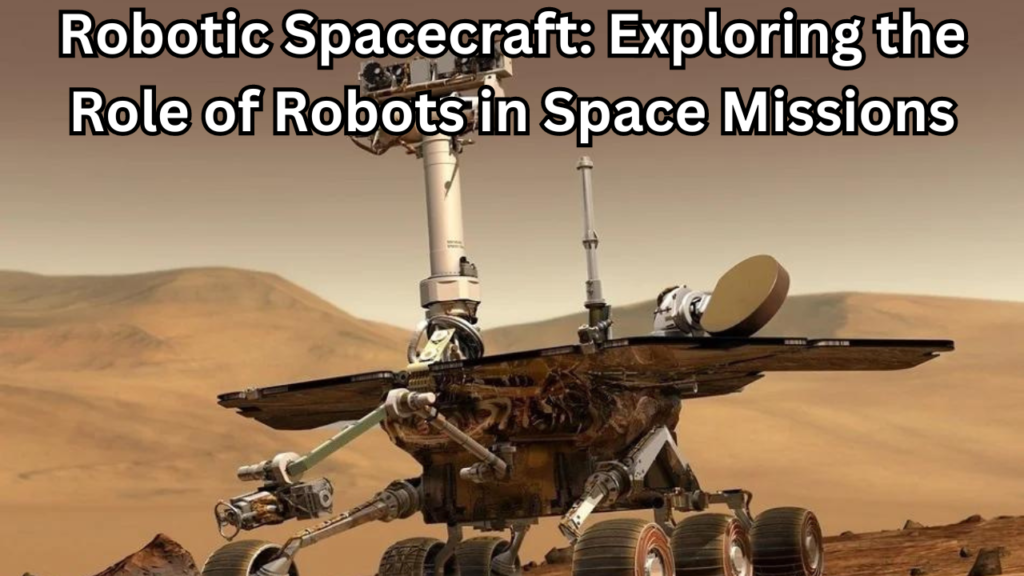
Table of Contents
Introduction
Robotic spacecraft have revolutionized our understanding of the universe, serving as our eyes and ears in the vast, uncharted territories of space. Unlike human missions, these automated explorers operate without the need for life support systems, making them ideal for tasks that are too hazardous or impractical for astronauts. Their ability to perform complex tasks and relay valuable data back to Earth has expanded the boundaries of human knowledge and paved the way for future exploration. In this article, we will delve into the pivotal role of robotic spacecraft in space missions, exploring their history, technological advancements, and significant contributions to our understanding of the cosmos.
Overview of Robotic Spacecraft
Robotic spacecraft are unmanned vehicles designed to travel beyond Earth’s atmosphere and carry out a variety of scientific, exploratory, and observational tasks. These spacecraft come in various forms, including orbiters that circle planets or moons, landers that touch down on celestial surfaces, rovers that traverse alien landscapes, and probes that venture into deep space. Equipped with sophisticated instruments, they are capable of conducting experiments, taking high-resolution images, and transmitting data back to mission control. The design and functionality of these robots are tailored to their specific missions, allowing them to perform a range of activities from analyzing planetary atmospheres to mapping the surfaces of distant worlds.
Importance of Robots in Space Missions
The significance of robotic spacecraft in space exploration cannot be overstated. They provide crucial insights into areas of the cosmos that would otherwise be inaccessible or too risky for human missions. By sending robots to explore planets, moons, asteroids, and comets, scientists can gather data that enhances our understanding of the solar system’s formation, the potential for life elsewhere, and the dynamics of various celestial phenomena. Robotic missions are often the precursors to human exploration, offering detailed reconnaissance that helps plan future manned missions. Additionally, robots can operate in environments that are extremely harsh or distant, such as the surface of Mars or the vacuum of deep space, making them indispensable tools for expanding our reach and knowledge.
Brief History of Robotic Space Exploration
The history of robotic space exploration dates back to the early 20th century, with the first significant milestones achieved in the 1960s during the space race. The Soviet Union’s Luna 2 became the first human-made object to impact the Moon in 1959, marking a new era in space exploration. This was followed by the successful landing of Luna 9, which provided the first images of the lunar surface. The United States joined the race with its Mariner missions to Venus and Mars, paving the way for a series of successful planetary missions. The 1970s and 1980s saw the advent of spacecraft like Voyager 1 and 2, which ventured into interstellar space and provided groundbreaking data about the outer planets and their moons. More recent decades have seen the deployment of sophisticated rovers like Spirit, Opportunity, and Curiosity on Mars, as well as missions like Cassini, which studied Saturn and its rings. Each of these milestones represents a leap forward in our ability to explore and understand the universe through robotic means, showcasing the ever-evolving capabilities of these remarkable machines.
The Evolution of Robotic Spacecraft
Early Developments and Milestones
The dawn of robotic Space Tech began in the late 1950s and early 1960s, a period marked by rapid technological advancements and ambitious space missions. The Soviet Union’s Luna program was among the first to achieve significant milestones, with Luna 1 in 1959 becoming the first spacecraft to reach the vicinity of the Moon, though it missed its target and instead entered orbit around the Sun. The subsequent successful impact of Luna 2 on the Moon’s surface marked humanity’s first physical contact with another celestial body. The United States soon followed with the Mariner program, with Mariner 2’s successful flyby of Venus in 1962 providing the first data on another planet’s atmosphere and temperature. These early missions set the stage for future robotic explorers, demonstrating the potential of unmanned spacecraft to gather invaluable data and achieve groundbreaking scientific discoveries.
Technological Advancements Over the Decades
As space exploration progressed, technological advancements significantly enhanced the capabilities of robotic spacecrafts. The 1970s introduced more sophisticated design and engineering approaches, with improved propulsion systems and better communication technologies. The Voyager missions, launched in 1977, exemplified this leap forward, utilizing advanced instruments to perform long-duration interstellar exploration. The 1980s and 1990s saw further refinements, including the development of more durable and versatile spacecraft. Innovations such as miniaturized sensors, enhanced imaging systems, and advanced autonomous navigation systems became standard. The advent of digital technology and miniaturization in the 2000s allowed for even more sophisticated missions, such as the Mars rovers Spirit and Opportunity, which featured advanced scientific instruments and high-resolution cameras. These advancements have continually pushed the boundaries of what robotic spacecraft can achieve, enabling deeper exploration and more detailed observations of distant worlds.
Key Missions and Their Impact
Several key missions have been pivotal in advancing our understanding of space and showcasing the potential of robotic spacecraft. The Mariner 4 mission, which successfully flew by Mars in 1965, provided the first close-up images of the Red Planet, revealing a barren and cratered surface. The Viking missions of the 1970s further expanded our knowledge of Mars by conducting surface experiments and searching for signs of life. The Voyager 1 and 2 missions, launched in 1977, provided unprecedented data on the outer planets and are now exploring the boundaries of the solar system. The Hubble Space Telescope, launched in 1990, has revolutionized our view of the universe with its high-resolution images of distant galaxies and nebulae. More recently, the Curiosity rover, landing on Mars in 2012, has provided critical information about the planet’s geology and climate, enhancing our understanding of its potential to support life. Each of these missions has had a profound impact on science, providing new insights into the nature of our solar system and beyond, and highlighting the essential role of robotic spacecraft in space exploration.
Types of Robotic Spacecraft
Orbiters
Function and Design
Orbiters are robotic spacecraft designed to enter and remain in orbit around a celestial body, such as a planet, moon, or asteroid. Their primary function is to capture high-resolution images, collect scientific data, and map the target body’s surface and atmosphere from orbit. The design of orbiters emphasizes durability and longevity, as they must operate for extended periods in space. Key components include advanced imaging systems, scientific instruments for measuring atmospheric composition and surface conditions, and propulsion systems that enable precise orbital adjustments. Orbiters are equipped with communication systems to transmit data back to Earth, and their stability in orbit is maintained by a combination of gyroscopes and attitude control systems.
Notable Orbiter Missions
Several missions have demonstrated the capabilities and contributions of orbiters. The Mars Reconnaissance Orbiter, launched in 2005, has provided detailed images of the Martian surface and atmosphere, enhancing our understanding of Mars’ geology and climate. The Galileo spacecraft, which orbited Jupiter from 1995 to 2003, offered unprecedented insights into the gas giant’s atmosphere and its moons, including detailed studies of Europa, which is believed to have a subsurface ocean. The Cassini-Huygens mission, which orbited Saturn from 2004 to 2017, delivered a wealth of information about Saturn’s rings and moons, including the discovery of water-ice geysers on Enceladus. These missions have significantly expanded our knowledge of the solar system and demonstrated the vital role of orbiters in space exploration.
Landers
Function and Design
Landers are robotic spacecraft designed to land on the surface of a celestial body and conduct scientific investigations directly at the landing site. Their primary functions include analyzing soil and rock samples, measuring environmental conditions, and conducting experiments that are not possible from orbit. The design of landers focuses on safe and precise landing capabilities, with features such as landing legs, descent and landing systems, and onboard laboratories for scientific analysis. Landers often include instruments for taking soil and atmospheric samples, cameras for capturing detailed images, and communication systems to relay data back to mission control.
Successful Lander Missions
Notable successful lander missions include the Viking landers, which touched down on Mars in 1976 and conducted a series of experiments to analyze Martian soil and search for signs of life. The Lunar Module, used during the Apollo missions, successfully landed astronauts on the Moon and provided critical information about the lunar surface. More recently, the Chang’e 3 mission, which landed on the Moon in 2013, deployed the Yutu rover and conducted valuable geological studies. The InSight lander, which landed on Mars in 2018, has provided significant data on the planet’s seismic activity and internal structure. These missions have offered direct insights into the surface conditions and composition of their target bodies, contributing to our understanding of planetary science.
Rovers
Function and Design
Rovers are robotic vehicles designed to traverse the surface of a celestial body, allowing for detailed exploration and analysis of varied terrain. They are equipped with a range of scientific instruments and tools to conduct experiments, analyze soil and rock samples, and capture images of the environment. The design of rovers includes mobility systems such as wheels or tracks for movement, a robust suspension system to handle uneven terrain, and a suite of scientific instruments for data collection. Rovers are also equipped with communication systems to transmit data back to Earth and navigation systems to plan and execute travel routes across the surface.
Famous Rover Missions
Some of the most famous rover missions include the Mars rovers Spirit and Opportunity, which landed on Mars in 2004 and conducted extensive geological surveys and exploration. The Curiosity rover, which landed on Mars in 2012, has been instrumental in studying the planet’s climate, geology, and potential habitability. The Perseverance rover, which landed on Mars in 2021, is tasked with searching for signs of ancient life and collecting samples for future return missions. The Sojourner rover, which was part of NASA’s Mars Pathfinder mission in 1997, was the first successful Mars rover and demonstrated the feasibility of mobile exploration on the Red Planet. These missions have provided invaluable data and expanded our knowledge of Mars and other celestial bodies.
Probes
Function and Design
Probes are robotic spacecraft designed to travel through space and perform scientific measurements of distant celestial objects, including planets, moons, asteroids, and comets. Unlike orbiters or landers, probes are often designed for flybys or short-term observations rather than long-term missions. Their primary function is to gather data about the target body’s composition, atmosphere, and other properties. The design of probes typically includes scientific instruments for data collection, communication systems for transmitting findings back to Earth, and propulsion systems for maneuvering through space. Some probes are also equipped with entry systems for studying atmospheres or surfaces during atmospheric entry.
Significant Probe Missions
Significant probe missions include the Voyager 1 and 2 missions, which launched in 1977 and provided groundbreaking data on the outer planets and are now exploring interstellar space. The New Horizons probe, which flew by Pluto in 2015, delivered the first close-up images and data of the dwarf planet, revealing its complex surface and atmosphere. The Rosetta mission, which included the Philae lander, successfully studied the comet 67P/Churyumov-Gerasimenko and provided insights into the composition and behavior of comets. The Juno probe, which entered orbit around Jupiter in 2016, has been studying the gas giant’s atmosphere and magnetic field, contributing to our understanding of its formation and evolution. These missions have made significant contributions to space science and exploration by providing detailed observations of distant and diverse celestial objects.
Mission Planning and Execution
Mission Objectives and Goals
Mission objectives and goals are fundamental in guiding the design and execution of robotic spacecraft missions. These objectives define the purpose of the mission, the scientific questions to be addressed, and the data to be collected. Objectives can range from studying the surface composition of a planet, analyzing atmospheric conditions, or investigating the potential for life on other worlds. Clear goals help determine the type of spacecraft required, the instruments to be included, and the mission’s overall strategy. For example, a mission to Mars might aim to explore its geology and search for signs of past life, while a mission to a distant comet might focus on understanding its composition and behavior. Establishing precise objectives ensures that all aspects of the mission are aligned and that the spacecraft is equipped to achieve its intended outcomes.
Designing Robotic Spacecraft for Specific Missions
Designing robotic spacecraft for specific missions involves tailoring the spacecraft’s features and capabilities to meet the unique requirements of the mission objectives. This process begins with defining the mission’s scientific goals and then translating these into technical specifications. For planetary missions, spacecraft design must consider factors such as the target body’s environment, whether it is an atmosphere, surface composition, or temperature extremes. For instance, a lander designed for Mars needs robust landing systems to handle the planet’s surface conditions, while a spacecraft heading to the outer planets requires powerful propulsion systems and long-lasting power sources. The design phase also involves selecting appropriate scientific instruments, communication systems for data transmission, and thermal controls to maintain optimal operating conditions. By customizing the design to meet the specific demands of the mission, engineers can maximize the spacecraft’s effectiveness and ensure that it successfully achieves its objectives.
Pre-Launch Preparations
Pre-launch preparations are crucial to ensure the successful deployment and operation of robotic spacecraft. This phase involves a series of rigorous tests and checks to verify that the spacecraft is ready for the harsh conditions in space travel. Key activities include assembly and integration, where the spacecraft’s various components and systems are assembled and tested together. Engineers conduct a series of performance tests to ensure that all systems, including propulsion, communication, and scientific instruments, function correctly. Thermal vacuum tests simulate the extreme temperatures and vacuum of space to confirm that the spacecraft can withstand these conditions. Additionally, pre-launch preparations include mission rehearsals and final checks to verify that all systems are operational and that the spacecraft is properly configured for launch. These thorough preparations are essential for mitigating risks and ensuring that the spacecraft can successfully complete its mission.
Launch and Deployment
The launch and deployment phase is the critical moment when the robotic spacecraft is sent on its journey to its target. This process begins with the spacecraft being transported to the launch site and mounted on a launch vehicle, such as a rocket. The launch vehicle’s role is to provide the necessary thrust to propel the spacecraft out of Earth’s atmosphere and into space. Once the rocket is launched, the spacecraft is carefully monitored as it ascends and reaches its designated trajectory. After launch, the spacecraft is deployed into its intended orbit or trajectory, and any necessary initial adjustments are made to ensure it is on the correct path. The deployment phase also includes the activation of onboard systems, such as communication links with mission control and the initial calibration of scientific instruments. This phase is critical for setting the spacecraft on its course and preparing it for the operational phase of its mission.
Key Components of Robotic Spacecraft
Propulsion Systems
Propulsion systems are a fundamental component of robotic spacecraft, responsible for maneuvering and controlling the spacecraft’s trajectory throughout its mission. These systems enable spacecraft to enter and maintain orbit, travel to distant celestial bodies, and execute precise maneuvers for scientific observations or landing operations. The choice of propulsion system depends on the mission’s requirements and the spacecraft’s destination. Chemical propulsion systems, such as liquid or solid rocket engines, provide high thrust for launches and significant orbital changes. For deep space missions, ion thrusters or electric propulsion systems offer greater efficiency and fuel economy, though with lower thrust. Additionally, reaction control systems, including thrusters and gyroscopes, help maintain the spacecraft’s orientation and stability. The design and integration of propulsion systems are crucial for achieving mission objectives and ensuring the spacecraft can navigate the complexities of space travel.
Communication Systems
Communication systems are essential for maintaining contact between the robotic spacecraft and mission control on Earth. These systems enable the transmission of data, including scientific observations, status updates, and commands for operational adjustments. The primary components of communication systems include high-gain antennas, which provide the necessary bandwidth for transmitting large volumes of data over long distances, and low-gain antennas, which facilitate basic communication during close-range operations. Spacecraft communication systems also include transponders and receivers that handle signal encoding, decoding, and error correction. Given the vast distances involved, communication systems must be robust and capable of handling signal delays and loss of data. Advanced technologies, such as deep space networks, ensure reliable and continuous communication with spacecraft, even as they travel far beyond Earth’s orbit.
Power Systems
Power systems are critical for providing the necessary energy to operate the various components of a robotic spacecraft. These systems ensure that the spacecraft’s scientific instruments, communication systems, propulsion units, and onboard computers have a consistent and reliable power supply. Solar panels are commonly used to generate electricity by harnessing sunlight, which is then converted into electrical power. In deep space missions where sunlight is limited, spacecraft may rely on radioisotope thermoelectric generators (RTGs) to produce power from the heat generated by the decay of radioactive isotopes. Power systems also include batteries for storing energy and managing power distribution. Effective power management is essential for maintaining spacecraft operations and extending mission duration, especially during periods when the spacecraft is in the shadow of celestial bodies or traveling through the dark regions of space.
Scientific Instruments and Sensors
Scientific instruments and sensors are the tools that enable robotic spacecraft to gather and analyze data about their target environments. These instruments are tailored to the specific objectives of the mission and can include a wide range of technologies. Cameras and imaging systems capture high-resolution pictures of planetary surfaces, moons, and other celestial phenomena. Spectrometers analyze the composition of materials by measuring light spectra, while mass spectrometers and chromatographs assess the presence of chemical elements and compounds. Other instruments, such as seismometers, measure geological activity, and magnetometers detect magnetic fields. Thermal sensors monitor temperature variations, and particle detectors study cosmic radiation and charged particles. The integration of these scientific instruments allows spacecraft to conduct detailed investigations, contributing to our understanding of space and the characteristics of other worlds.
Navigation and Control
Autonomous Navigation
Autonomous navigation is a crucial capability for robotic spacecraft, enabling them to independently maneuver and adjust their trajectories without direct intervention from ground control. This system relies on onboard sensors, cameras, and algorithms to detect and interpret the spacecraft’s surroundings, allowing it to make real-time decisions and execute complex maneuvers. Autonomous navigation is particularly important for missions where communication delays with Earth can be substantial, such as those involving deep space or planetary landings. Technologies like star trackers, inertial measurement units, and optical navigation systems help the spacecraft determine its position and orientation with high precision. By utilizing these systems, spacecraft can perform tasks such as avoiding obstacles, adjusting orbits, and executing landing sequences autonomously, enhancing mission efficiency and reliability.
Ground Control and Remote Operations
Ground control and remote operations are essential for managing and overseeing the activities of robotic spacecraft from Earth. Ground control teams are responsible for sending commands, monitoring spacecraft status, and analyzing data received from the mission. This involves operating mission control centers equipped with advanced communication and data processing facilities. Remote operations enable ground-based engineers and scientists to adjust spacecraft parameters, plan scientific experiments, and troubleshoot issues as they arise. The coordination between ground control and spacecraft is facilitated by sophisticated communication systems that relay information across vast distances. This interaction ensures that the spacecraft remains on course, performs its intended functions, and collects valuable scientific data, despite being millions of miles away from Earth.
Challenges in Spacecraft Navigation
Spacecraft navigation presents several challenges due to the complexities of space travel and the extreme environments encountered. One significant challenge is the vast distances involved, which can result in communication delays of several minutes to hours, making real-time control difficult. Additionally, spacecraft must navigate through unpredictable space environments, such as asteroid fields or the radiation belts of planets, which can affect their trajectory and operational systems. Precision is also crucial, as small errors in navigation can lead to significant deviations from the intended path, potentially jeopardizing mission success. Furthermore, spacecraft must contend with the effects of microgravity and lack of atmospheric reference points, complicating the task of maintaining accurate orientation and positioning. Advanced navigation technologies and robust error-correction algorithms are continually developed to address these challenges and ensure successful mission execution.
Scientific Contributions
Planetary Exploration
Planetary exploration involves sending robotic spacecraft to study the various planets, moons, and other celestial bodies within our solar system. This exploration aims to understand the formation, composition, and dynamics of these bodies, as well as their potential for hosting life. Robotic missions can include orbiters that survey planets from space, landers that touch down on surfaces, and rovers that traverse planetary terrains to collect samples and conduct experiments. The data gathered from these missions help scientists unravel the history of our solar system, examine planetary atmospheres, and assess surface conditions. Advances in technology and instrumentation have greatly enhanced our ability to explore and understand the diverse environments of these celestial bodies.
Mars Missions
Mars missions have been some of the most ambitious and high-profile endeavors in planetary exploration. These missions aim to investigate the Red Planet’s geology, climate, and potential for past or present life. Notable missions include the Mars rovers Spirit and Opportunity, which conducted extensive geological surveys and provided insights into Mars’ surface history. The Curiosity rover, which landed in 2012, has been analyzing Martian rocks and soil to assess the planet’s habitability. The Perseverance rover, which landed in 2021, is focused on searching for signs of ancient life and collecting samples for future return missions. Mars missions have significantly advanced our understanding of the planet’s potential to support life and its suitability for future human exploration.
Lunar Missions
Lunar missions aim to explore Earth’s Moon, providing insights into its geology, composition, and history. The Apollo missions of the 1960s and 1970s were pivotal, as they not only landed astronauts on the Moon but also returned samples that have been crucial for scientific study. More recent missions, such as NASA’s Lunar Reconnaissance Orbiter and China’s Chang’e program, have provided detailed maps of the lunar surface and investigated the presence of water ice in the polar regions. These missions help us understand the Moon’s role in the history of the solar system, its geological processes, and its potential for future exploration and resource utilization.
Missions to Other Planets
Exploration of planets beyond Mars includes missions to Venus, Jupiter, Saturn, and their moons. For example, the Venus Express mission provided valuable data on Venus’s atmospheric composition and weather patterns. The Galileo spacecraft orbited Jupiter and conducted detailed studies of its moons, including Europa, which is believed to have a subsurface ocean. The Cassini spacecraft, which orbited Saturn, offered groundbreaking insights into the planet’s rings and moons, including the discovery of geysers on Enceladus. These missions expand our understanding of planetary systems and the diverse environments within our solar system, contributing to a broader knowledge of planetary science and potential habitats.
Astrobiology and the Search for Life
Astrobiology is the study of life in the universe, focusing on the potential for life beyond Earth and the conditions that might support it. Robotic missions are instrumental in this search, analyzing extraterrestrial environments for signs of life or conditions conducive to life. For example, missions to Mars aim to detect biomarkers and analyze surface and atmospheric samples for signs of past or present life. Similarly, missions to icy moons like Europa and Enceladus investigate subsurface oceans that might harbor life. Astrobiology also involves studying extreme environments on Earth to understand how life might survive in harsh conditions elsewhere in the universe. These efforts are crucial for answering fundamental questions about the existence of life beyond our planet and the potential for discovering habitable environments.
Astrophysics and Cosmology
Astrophysics and cosmology explore the fundamental aspects of the universe, including its origins, structure, and evolution. Astrophysics focuses on understanding the physical properties and behaviors of celestial objects, such as stars, galaxies, and black holes. Cosmology, on the other hand, studies the large-scale structure and history of the universe, including the Big Bang and the expansion of the cosmos. Robotic spacecraft and observatories play a key role in these fields by providing detailed observations and data from space. Instruments such as the Hubble Space Telescope have revolutionized our understanding of the universe, offering deep insights into the formation of galaxies and the nature of dark matter. These studies contribute to a broader understanding of the universe’s fundamental properties and the processes that govern its evolution.
Robotic Spacecraft in Asteroid and Comet Missions
Importance of Studying Small Celestial Bodies
Studying small celestial bodies, such as asteroids and comets, provides crucial insights into the early solar system and the formation of planetary systems. These objects are remnants from the early solar system and offer a snapshot of the conditions that existed billions of years ago. By analyzing their composition, structure, and behavior, scientists can better understand the building blocks of planets and the processes that shaped the solar system. Small celestial bodies can also serve as analogs for understanding the potential for similar objects elsewhere in the galaxy. Additionally, studying these bodies can help assess potential hazards they might pose to Earth, as well as explore their potential as resources for future space exploration and mining. Overall, small celestial bodies are valuable targets for scientific research and practical applications in space exploration.
Notable Missions to Asteroids and Comets
Several notable missions have significantly advanced our knowledge of asteroids and comets. The Near-Earth Asteroid Rendezvous (NEAR) mission, which orbited and landed on the asteroid Eros in 2001, provided detailed data on the asteroid’s surface composition and structure. NASA’s OSIRIS-REx mission, launched in 2016, successfully collected samples from the asteroid Bennu and is returning them to Earth for analysis, offering insights into the asteroid’s origins and its potential role in the early solar system. The European Space Agency’s Rosetta mission, which included the Philae lander, successfully orbited and landed on the comet 67P/Churyumov-Gerasimenko, providing unprecedented data on cometary composition and behavior. These missions have expanded our understanding of these small celestial bodies, revealing details about their physical properties, chemical makeup, and dynamic processes.
Discoveries and Insights
The study of small celestial bodies has led to several groundbreaking discoveries and insights. For instance, the NEAR mission revealed that the asteroid Eros is composed of a mixture of rock and metal, suggesting a complex history of collisional and accretionary processes. The OSIRIS-REx mission’s sample return from Bennu is expected to provide new information about the asteroid’s organic compounds and minerals, potentially offering clues about the origins of life on Earth. Rosetta’s observations of comet 67P revealed a complex surface with diverse terrains, including active outgassing and water ice, shedding light on the processes that drive cometary activity. These discoveries not only enhance our understanding of the early solar system but also offer valuable data for assessing the potential for future exploration and resource utilization of these celestial bodies.
Spacecraft in Deep Space Exploration
Voyager Missions
The Voyager missions, launched by NASA in 1977, are among the most iconic and influential space missions in the history of space exploration. Voyager 1 and Voyager 2 were designed to explore the outer planets of our solar system and continue their journey into interstellar space. Voyager 1’s primary mission included flybys of Jupiter and Saturn, providing stunning images and valuable data about these gas giants and their moons. Voyager 2, the only spacecraft to visit all four of the solar system’s giant planets—Jupiter, Saturn, Uranus, and Neptune—offered a comprehensive view of the outer planets and their complex systems. Both spacecraft are equipped with scientific instruments that have contributed to our understanding of planetary atmospheres, magnetospheres, and ring systems. Voyager 1, currently the farthest human-made object from Earth, has entered interstellar space, providing unprecedented data on the boundary between the solar system and interstellar space.
New Horizons and Beyond
The New Horizons mission, launched in 2006, was a landmark in the exploration of the outer solar system. Its primary mission was to conduct a flyby of Pluto, the first close-up study of the dwarf planet, and its moons. New Horizons provided detailed images and data on Pluto’s surface, revealing its complex geology, including icy plains, mountain ranges, and a hazy atmosphere. After its successful encounter with Pluto in 2015, New Horizons continued its journey into the Kuiper Belt, a region of the solar system beyond Neptune populated by small icy bodies. In 2019, the spacecraft conducted a flyby of Arrokoth (formerly known as Ultima Thule), a Kuiper Belt object, offering insights into the early solar system’s formation. New Horizons’ mission has expanded our understanding of the outer solar system and provided a wealth of data on distant and previously unexplored regions.
The Future of Deep Space Probes
The future of deep space probes promises to further expand our exploration capabilities and enhance our understanding of the solar system and beyond. Upcoming missions are likely to focus on exploring more distant and challenging targets, such as the outermost reaches of the solar system, interstellar space, and exoplanets. Advancements in propulsion technology, such as nuclear thermal propulsion and ion drives, could enable faster and more efficient travel to these distant destinations. New missions may include advanced spacecraft designed to study the interstellar medium, search for signs of extraterrestrial life, and investigate the nature of dark matter and dark energy. Innovations in communication technology will be crucial for maintaining contact with these distant probes, ensuring data transmission across vast distances. As technology evolves and our scientific goals become more ambitious, future deep space probes will continue to push the boundaries of human exploration and discovery.
Robotic Spacecraft in Earth Observation
Monitoring Climate and Weather Patterns
Monitoring climate and weather patterns is a vital application of satellite technology, providing crucial data for understanding and predicting atmospheric conditions. Satellites equipped with advanced sensors and instruments continuously observe various parameters such as temperature, humidity, wind speeds, and cloud cover. This data helps scientists track long-term climate changes, such as global warming and shifts in weather patterns. By analyzing satellite observations, researchers can identify trends in climate variables, monitor greenhouse gas concentrations, and assess the impacts of climate change on different regions. Additionally, satellites play a key role in weather forecasting by providing real-time data on storm systems, precipitation, and atmospheric pressure. This information supports early warning systems and enhances our ability to predict and prepare for extreme weather events, contributing to better disaster preparedness and mitigation efforts.
Tracking Natural Disasters
Satellites are invaluable tools for tracking and managing natural disasters, offering real-time monitoring and detailed observations of events such as hurricanes, earthquakes, floods, and wildfires. During a hurricane, satellites provide crucial data on storm intensity, structure, and movement, enabling accurate forecasts and tracking of the storm’s progress. For earthquakes, while satellites do not detect seismic activity directly, they monitor ground deformation and changes in land surface, which can help assess the impact of seismic events and guide emergency response efforts. In the case of floods and wildfires, satellites can capture high-resolution images and thermal data to assess the extent of damage, monitor ongoing conditions, and support disaster response and recovery operations. The ability to observe and analyze these events from space enhances our understanding of their behavior and supports more effective disaster management strategies.
Environmental and Agricultural Applications
Environmental and agricultural applications of satellite technology offer significant benefits for managing natural resources and optimizing agricultural practices. Satellites provide valuable data on land use, vegetation health, and soil moisture, which are essential for monitoring and managing ecosystems. For agriculture, satellite imagery helps farmers and researchers assess crop health, predict yields, and manage irrigation. By analyzing data on vegetation cover and growth patterns, satellite technology can support precision farming techniques, optimize resource use, and improve crop productivity. Additionally, satellites contribute to environmental conservation efforts by monitoring deforestation, tracking changes in biodiversity, and assessing the impact of human activities on natural habitats. Overall, the integration of satellite data into environmental and agricultural management enhances our ability to make informed decisions and promote sustainable practices.
Collaborative International Missions
Partnerships Between Space Agencies
Partnerships between space agencies are crucial for advancing space exploration and scientific research. Collaborative efforts among international space agencies allow for the pooling of resources, expertise, and technologies, which can enhance mission capabilities and reduce costs. Major space agencies, such as NASA (United States), ESA (European Space Agency), Roscosmos (Russia), CNSA (China National Space Administration), and JAXA (Japan Aerospace Exploration Agency), often collaborate on missions that require a range of specialized skills and resources. These partnerships enable the sharing of data, joint development of spacecraft and instruments, and coordinated mission planning. By working together, space agencies can tackle more ambitious projects, address complex scientific questions, and achieve milestones that might be challenging for a single agency to accomplish alone. Collaborative efforts also foster international goodwill and promote peaceful uses of outer space.
Joint Ventures and Their Achievements
Joint ventures between space agencies have led to numerous significant achievements in space exploration. One prominent example is the International Space Station (ISS), a collaborative project involving NASA, ESA, Roscosmos, JAXA, and the Canadian Space Agency (CSA). The ISS serves as a microgravity laboratory for scientific research and international cooperation, contributing to our understanding of space science, technology development, and life sciences. Another notable joint venture is the Mars Science Laboratory mission, which saw collaboration between NASA and the European Space Agency. This mission included the Mars Curiosity rover, which has provided valuable data about the Martian surface. Additionally, the Hubble Space Telescope, developed in collaboration between NASA and ESA, has revolutionized our understanding of the universe with its high-resolution images and data. These joint ventures demonstrate the power of international cooperation in achieving complex and groundbreaking space missions.
The Role of International Collaboration
International collaboration plays a vital role in the advancement of space exploration and research. By working together, countries can leverage their collective strengths, including technological expertise, financial resources, and scientific knowledge. This collaboration helps to mitigate risks, share costs, and address challenges that are beyond the scope of individual nations. It also fosters the exchange of ideas and promotes innovation by bringing together diverse perspectives and approaches. International partnerships can lead to the development of new technologies, enhance scientific discoveries, and accelerate progress in space exploration. Furthermore, these collaborations contribute to the peaceful use of outer space and strengthen global cooperation in addressing shared challenges, such as space debris management and planetary defense. Overall, international collaboration is essential for advancing human knowledge and achieving the long-term goals of space exploration.
The Future of Robotic Spacecraft
Upcoming Missions and Innovations
Upcoming missions and innovations in space exploration are set to push the boundaries of our understanding of the cosmos and our capabilities in space travel. Notable missions on the horizon include NASA’s Artemis program, which aims to return humans to the Moon by the mid-2020s and establish a sustainable presence for future exploration. This program will lay the groundwork for deeper space missions, including potential manned missions to Mars. The European Space Agency’s Jupiter Icy Moons Explorer (JUICE) mission is set to launch in 2023 with the goal of exploring Jupiter’s moons, particularly Europa, Ganymede, and Callisto, to investigate their potential for supporting life. Innovations such as advanced propulsion systems, including nuclear thermal and electric propulsion, are expected to enhance the efficiency and speed of space travel. Additionally, the development of new technologies like autonomous spacecraft and advanced robotics will enable more complex and precise missions. These advancements will drive the next era of space exploration, offering new opportunities for scientific discovery and human expansion into the solar system.
The Role of AI and Machine Learning
Artificial intelligence (AI) and machine learning are increasingly playing a crucial role in space exploration, transforming how missions are planned, executed, and analyzed. AI algorithms can process and analyze vast amounts of data collected by spacecraft and telescopes, identifying patterns and anomalies that might be missed by human researchers. Machine learning models are used to improve image processing, enhance navigation systems, and optimize mission operations. For instance, AI-driven systems can autonomously detect and avoid obstacles, adjust spacecraft trajectories, and manage resource allocation. In mission planning, AI can simulate various scenarios and predict outcomes, helping engineers design more robust and efficient spacecraft. Additionally, AI is instrumental in analyzing data from space missions, such as identifying exoplanets or analyzing surface samples from distant planets. The integration of AI and machine learning into space exploration enhances our ability to explore and understand the universe while improving the efficiency and capabilities of space missions.
Future Prospects for Interstellar Travel
The future prospects for interstellar travel are an exciting and ambitious area of research, aiming to extend human exploration beyond our solar system. Current theoretical concepts for interstellar travel include advanced propulsion technologies, such as nuclear fusion, antimatter drives, and light sails. These technologies aim to achieve the high velocities necessary to reach nearby star systems within a human lifetime. Projects like the Breakthrough Starshot initiative seek to develop lightweight, high-speed spacecraft propelled by laser beams, potentially allowing for travel to the closest stars within a few decades. Additionally, advancements in propulsion systems, materials science, and energy generation are crucial for making interstellar travel feasible. While practical interstellar missions are still in the conceptual and experimental stages, continued research and innovation in these areas hold the promise of eventually enabling humanity to explore other star systems, significantly expanding our reach and understanding of the cosmos.
Challenges and Limitations
Technical and Engineering Hurdles
Technical and engineering hurdles are significant challenges in the field of space exploration, requiring innovative solutions and extensive testing to overcome. One major hurdle is the development of advanced propulsion systems capable of achieving the high speeds necessary for deep space travel. Current propulsion technologies are limited by efficiency and speed, necessitating research into alternative methods such as nuclear propulsion or ion drives. Additionally, spacecraft must be designed to withstand the harsh conditions of space, including extreme temperatures, radiation, and micrometeoroid impacts. Engineering reliable and durable systems that can function over long periods in the vacuum of space is a complex task. Another challenge is ensuring effective communication over vast distances, which requires robust data transmission technologies and error-correction algorithms. These technical and engineering hurdles demand significant resources, interdisciplinary expertise, and rigorous testing to ensure mission success and safety.
Budget Constraints
Budget constraints are a critical issue in space exploration, influencing the scope and feasibility of missions. Developing and launching spacecraft involves substantial costs, including research and development, manufacturing, testing, and operational expenses. Limited funding can restrict the scope of missions, delay project timelines, or lead to the cancellation of ambitious projects. Space agencies and organizations must prioritize their objectives and allocate resources effectively to balance scientific goals with budgetary limitations. Collaborative efforts between countries and private sector partnerships can help mitigate these constraints by sharing costs and leveraging additional funding sources. Furthermore, the development of cost-effective technologies and efficient project management practices can help optimize the use of available funds. Addressing budget constraints is essential for sustaining long-term space exploration programs and achieving significant scientific and exploratory milestones.
Ethical Considerations
Ethical considerations are increasingly important in space exploration as missions become more complex and impactful. One key ethical issue is the potential contamination of extraterrestrial environments. There is a responsibility to prevent biological contamination of other planets or moons, which could interfere with scientific studies and potentially harm any existing ecosystems. Planetary protection protocols are in place to address this concern, but ongoing vigilance and adherence to these guidelines are necessary. Additionally, the potential exploitation of space resources raises ethical questions about the ownership and utilization of extraterrestrial materials. As private companies and nations seek to mine asteroids or establish lunar bases, it is important to consider the legal and ethical implications of these activities. Furthermore, space exploration involves significant investments and risks, prompting discussions about the allocation of resources and the potential benefits to humanity. Addressing these ethical considerations thoughtfully and collaboratively is crucial for ensuring responsible and sustainable space exploration practices.
Conclusion
Recap of Key Points
Robotic spacecraft have revolutionized our understanding of the universe by providing critical data from distant and often inaccessible regions of space. These spacecraft have played a pivotal role in planetary exploration, with missions to Mars, the Moon, and beyond expanding our knowledge of celestial bodies and their potential to support life. Technological advancements in propulsion, communication, and autonomous navigation have enhanced the capabilities of these missions, enabling more complex and ambitious projects. Key missions, such as the Voyager probes and the Mars rovers, have delivered groundbreaking discoveries and insights about the solar system and interstellar space. The collaboration between international space agencies has been instrumental in achieving these milestones, showcasing the benefits of shared expertise and resources. As we look forward, the integration of AI and machine learning will further enhance mission efficiency and scientific outcomes, while future prospects for interstellar travel promise to extend our reach beyond the solar system.
The Continued Importance of Robotic Spacecraft
The continued importance of robotic spacecraft lies in their ability to explore and gather data from environments that are currently beyond human reach. These spacecraft are essential for conducting detailed scientific investigations of distant planets, moons, and other celestial objects. They offer invaluable information about the composition, structure, and conditions of these bodies, which is crucial for understanding the formation and evolution of the solar system. Moreover, robotic missions are more cost-effective and safer than manned missions, allowing for extensive exploration with limited resources. As technology advances, robotic spacecraft will continue to play a central role in expanding our knowledge of the universe, including potential future missions to the outer edges of the solar system and beyond. Their ability to autonomously conduct experiments and gather data will remain fundamental to the progress of space exploration and science.
Looking Ahead to Future Missions
Looking ahead to future missions, the scope and ambition of space exploration are set to expand significantly. Upcoming missions will focus on exploring more distant and challenging targets, such as the outer planets, their moons, and the Kuiper Belt. Advances in propulsion technology, including nuclear and ion drives, will enable faster and more efficient travel to these far-reaching destinations. Missions planned for the coming decades include NASA’s Artemis program, aimed at returning humans to the Moon and establishing a sustainable presence, and the potential for crewed missions to Mars. Innovations in spacecraft design, such as enhanced autonomy and AI integration, will drive the success of these missions by improving navigation, data analysis, and operational efficiency. Additionally, future endeavors may involve interstellar probes that seek to explore the nearest star systems, pushing the boundaries of human exploration. The continued investment in research, technology, and international collaboration will be key to realizing these ambitious goals and unlocking new discoveries in the cosmos.
FAQs
What are Robotic Spacecraft?
Robotic spacecraft are unmanned vehicles designed to explore space and gather scientific data. Unlike crewed spacecraft, these robots are operated remotely from Earth or autonomously, using pre-programmed instructions and onboard systems to perform their missions. They are equipped with a range of instruments and sensors to capture images, analyze samples, and conduct experiments. Robotic spacecraft come in various forms, including orbiters, landers, rovers, and probes, each designed for specific tasks and environments. Their primary purpose is to explore and investigate celestial bodies, conduct scientific research, and provide valuable information about space phenomena and planetary conditions.
How do Robotic Spacecraft Contribute to Space Exploration?
Robotic spacecraft contribute to space exploration by performing tasks and gathering data that are crucial for understanding the cosmos. They explore planets, moons, asteroids, and comets, providing detailed information about their composition, structure, and behavior. This data helps scientists to study planetary atmospheres, surface geology, and potential for life, which are fundamental for understanding the formation and evolution of our solar system. Robotic missions also serve as precursors to human exploration, assessing the conditions and risks of new environments before sending astronauts. They can operate in extreme conditions and environments where human presence is impractical, making them essential for exploring distant or hazardous areas of space. Their findings support mission planning, inform scientific theories, and advance technology, contributing significantly to our knowledge of the universe.
What are Some Famous Robotic Space Missions?
Several robotic space missions have achieved significant milestones and provided groundbreaking discoveries. The Voyager missions, launched in 1977, explored the outer planets and continue to transmit data from interstellar space. The Mars rovers, including Spirit, Opportunity, and Curiosity, have explored the Martian surface, revealing evidence of past water activity and advancing our understanding of Mars. The Hubble Space Telescope, a joint NASA-ESA project, has revolutionized our view of the universe with its high-resolution observations. The Rosetta mission, with its Philae lander, provided the first detailed images of a comet’s surface and studied its composition. Additionally, the OSIRIS-REx mission successfully collected samples from the asteroid Bennu, offering insights into the building blocks of the solar system.
What Challenges Do Robotic Spacecraft Face?
Robotic spacecraft face several challenges during their missions. One major challenge is ensuring reliability and durability in the harsh environment of space, which includes extreme temperatures, radiation, and micrometeoroid impacts. Developing and maintaining robust systems that can operate autonomously for extended periods is complex and requires extensive testing. Communication delays and data transmission over vast distances pose another challenge, particularly for missions beyond the Moon. Robotic spacecraft must also be designed to handle the complexities of their scientific instruments and adapt to unexpected conditions. Additionally, the high cost of space missions necessitates careful planning and resource management to address technical issues and potential failures. Overcoming these challenges requires innovation, meticulous engineering, and thorough preparation.
What is the Future of Robotic Space Missions?
The future of robotic space missions is poised for exciting advancements and expanded exploration. Upcoming missions will focus on investigating more distant and challenging targets, such as the outer planets and their moons, as well as exploring the Kuiper Belt and potentially interstellar space. Technological innovations, such as advanced propulsion systems, autonomous navigation, and AI-driven data analysis, will enhance the capabilities and efficiency of these missions. Future robotic spacecraft may include more sophisticated landers and rovers capable of conducting complex scientific experiments and in-situ resource utilization. Additionally, collaborations between international space agencies and private companies will drive innovation and expand the scope of robotic missions. As technology progresses, robotic spacecraft will continue to play a crucial role in exploring the far reaches of the solar system and beyond, contributing to our understanding of the universe and laying the groundwork for future human exploration.