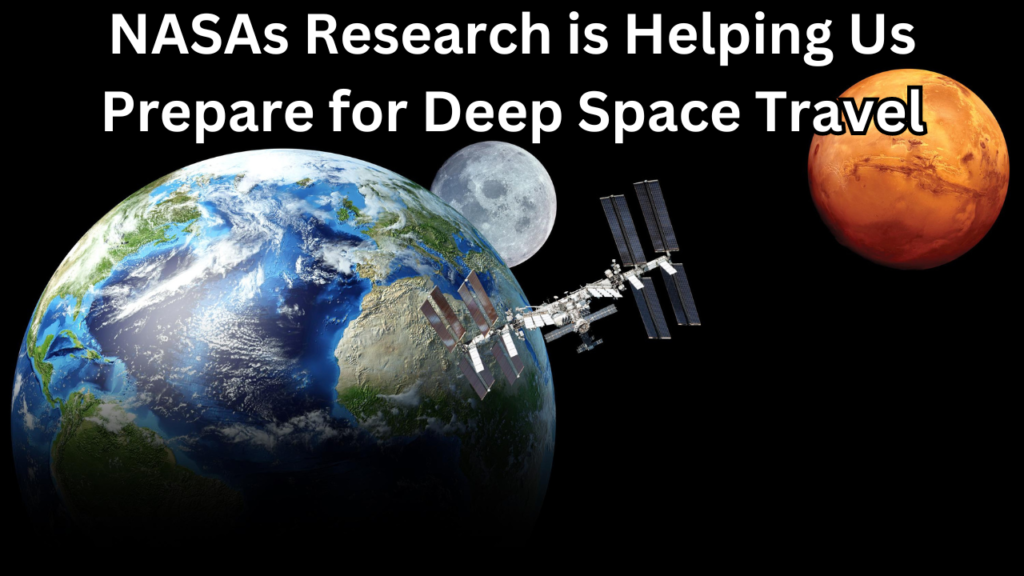
Table of Contents
Introduction
The Growing Ambition for Deep Space Exploration
Humanity’s quest to explore beyond our planet has evolved from the early days of space travel to an ambitious pursuit of deep space exploration. As technological advancements continue to progress, space agencies and private organizations are setting their sights on distant celestial bodies, such as Mars and the outer moons of our solar system. This ambition is driven by a combination of scientific curiosity, the desire to understand the origins of our universe, and the potential for discovering new resources and habitats beyond Earth.
NASAs Research is Helping Us Prepare for Deep Space Travel is no longer confined to theoretical discussions or distant dreams. It involves practical steps and technologies designed to overcome the immense challenges of traveling to and exploring regions far beyond Earth’s orbit. The focus has shifted from merely reaching low Earth orbit to developing capabilities that will enable humanity to live and work in the harsh environments of space for extended periods. This transition marks a significant leap in space exploration, with missions planned to venture into uncharted territories, testing the limits of human endurance and technological innovation.
Importance of NASA’s Research in Pioneering Deep Space Missions
NASA has been at the forefront of space exploration since the 1960s, and its role in pioneering deep space missions is crucial for several reasons. As a leading space agency, NASA’s research and development efforts are integral to advancing the technologies and knowledge necessary for successful deep space travel. The agency’s extensive experience in space exploration provides a foundation upon which future missions can be built.
NASA’s research encompasses a wide range of disciplines, including spacecraft design, propulsion systems, life support technologies, and mission planning. Through its various programs and initiatives, NASA is developing the necessary tools and technologies to address the complex challenges of deep space travel. This includes designing spacecraft that can withstand the harsh conditions of space, creating systems to support human life over long durations, and developing advanced propulsion methods to reach distant destinations more efficiently.
Moreover, NASA’s deep space missions are not isolated endeavors. They often involve collaboration with international space agencies, private companies, and research institutions. This collaborative approach ensures that the best minds and resources are dedicated to solving the intricate problems associated with deep space exploration. By setting ambitious goals and pursuing cutting-edge research, NASA is not only pushing the boundaries of what is possible but also laying the groundwork for future missions that will extend humanity’s reach into the farthest corners of the solar system and beyond.
Advancements in Spacecraft Technology
Development of the Orion Spacecraft
The Orion spacecraft represents a significant leap forward in spacecraft design and capability, developed by NASA to support deep space exploration missions. Designed to transport astronauts beyond low Earth orbit, Orion is a key component of NASA’s vision for human spaceflight to destinations such as the Moon and Mars. The spacecraft features a robust design that includes a heat shield capable of withstanding the intense re-entry temperatures of deep space travel, as well as a reinforced crew module to protect astronauts from the harsh conditions of space. Orion is equipped with advanced avionics and communication systems, ensuring reliable operation and connectivity throughout its missions.
Design and Capabilities
Orion’s design incorporates several advanced features to enhance its capabilities. It includes a service module provided by the European Space Agency (ESA), which supports propulsion, power generation, and thermal control. The spacecraft is designed to accommodate up to four astronauts on deep space missions and is equipped with life support systems to sustain crew members during extended periods. Orion’s multi-layered thermal protection system and radiation shielding are crucial for safeguarding the crew from space radiation and temperature extremes. Additionally, Orion’s modular design allows for compatibility with various launch systems and mission profiles, making it a versatile platform for future exploration.
Testing and Milestones
Orion has undergone a rigorous testing and development process to ensure its readiness for deep space missions. Key milestones include the successful completion of several uncrewed test flights, such as the Exploration Flight Test-1 (EFT-1) in 2014, which demonstrated the spacecraft’s performance and re-entry capabilities. Subsequent tests have focused on refining the spacecraft’s systems, including its propulsion and life support systems. The spacecraft’s first crewed mission, Artemis I, aims to validate Orion’s performance in actual space conditions and pave the way for future crewed missions to the Moon and beyond. These milestones are critical in validating Orion’s design and ensuring its reliability for deep space exploration.
Space Launch System (SLS)
The Space Launch System (SLS) is NASA’s new heavy-lift launch vehicle designed to propel spacecraft like Orion into deep space. It is the most powerful rocket ever built, with a thrust capability surpassing that of the Saturn V rockets used during the Apollo program. The SLS features a core stage with four RS-25 engines and two solid rocket boosters, providing the necessary power to lift heavy payloads into orbit. Its design includes advanced technologies to maximize efficiency and safety, ensuring that it can meet the demands of deep space missions.
Specifications and Power
The SLS is characterized by its impressive specifications, including a lift-off thrust of over 8.8 million pounds and a total height of approximately 322 feet. This immense power is derived from its combination of a highly efficient core stage and powerful solid rocket boosters. The rocket’s first stage utilizes the RS-25 engines, which have a proven track record from previous space shuttle programs, while the solid rocket boosters provide additional thrust during the initial phase of the launch. This powerful configuration enables the SLS to carry heavy payloads, including crewed spacecraft and scientific instruments, to destinations beyond low Earth orbit.
Launch Schedule and Objectives
The launch schedule for the SLS is strategically aligned with NASA’s deep space exploration goals. The first launch, known as Artemis I, is an uncrewed test mission designed to validate the SLS’s performance and its integration with the Orion spacecraft. Subsequent missions will progressively increase in complexity, with Artemis II planned as the first crewed mission to orbit the Moon and Artemis III targeting a lunar landing. The SLS will play a crucial role in these missions, providing the necessary lift and thrust to support human exploration of the Moon, Mars, and other deep space destinations. The rocket’s development and launch schedule are critical for achieving NASA’s long-term exploration objectives.
Habitation Modules
Habitation modules are essential components for supporting human life during extended space missions. These modules provide living quarters, workspaces, and necessary amenities to ensure astronauts’ comfort and safety. The design of these modules focuses on creating a functional and sustainable environment that can support crew members over long durations. This includes advanced life support systems for air and water recycling, as well as provisions for exercise, recreation, and medical care. The development of habitation modules is crucial for enabling deep space missions, as they ensure that astronauts have the necessary infrastructure to live and work effectively in space.
Deep Space Habitat Concepts
Deep space habitat concepts are being developed to address the unique challenges of long-duration missions beyond low Earth orbit. These habitats must be capable of sustaining life in the harsh environment of deep space, including protection from radiation and micrometeoroid impacts. Concepts include both pressurized and inflatable habitats, designed to be modular and expandable to accommodate varying mission requirements. These habitats are equipped with advanced life support systems, regenerative environmental controls, and communication systems to support crew members in maintaining their health and productivity during missions to the Moon, Mars, and beyond.
Inflatable Habitat Technology
Inflatable habitat technology represents an innovative approach to space habitat design. Unlike traditional rigid structures, inflatable habitats use lightweight materials that can be expanded once in space, offering a significant advantage in terms of launch efficiency and space utilization. These habitats are designed to be compact during launch and expand to provide larger living and working spaces once in orbit or on a planetary surface. Inflatable habitats incorporate advanced materials and structural design features to ensure durability and safety, including radiation shielding and thermal control. This technology holds promise for enhancing the flexibility and sustainability of deep space missions, providing astronauts with more comfortable and functional living environments.
Propulsion Systems for Deep Space
Chemical Propulsion
Chemical propulsion has been the cornerstone of space travel for decades, utilizing the reaction of chemical propellants to generate thrust. Traditional chemical rockets operate by burning a fuel and an oxidizer to produce high-pressure gases that are expelled through a nozzle, creating thrust in the opposite direction. This technology is well-understood and reliable, having powered landmark missions such as the Apollo Moon landings and Mars rover deployments. Advances in chemical propulsion focus on improving efficiency, increasing thrust-to-weight ratios, and extending the operational range of rocket engines. These improvements aim to enhance performance for both crewed and uncrewed missions, making chemical propulsion a continuing vital component of space exploration.
Traditional Rocket Propulsion Advances
Recent advancements in traditional rocket propulsion include the development of more efficient and powerful engines. Innovations such as the use of advanced composite materials and improved propellant formulations contribute to increased performance and reduced weight. For example, the Space Launch System (SLS) and its RS-25 engines are built on technologies derived from the Space Shuttle program, but with significant upgrades to increase thrust and reliability. Additionally, ongoing research into next-generation chemical engines focuses on enhancing specific impulse, which measures how efficiently a rocket uses propellant. These advances help make space missions more cost-effective and capable of carrying heavier payloads to higher orbits or deeper into space.
Current Projects and Innovations
Current projects and innovations in chemical propulsion include several notable initiatives aimed at pushing the boundaries of space travel. The development of reusable rocket technology by companies like SpaceX has revolutionized the field by significantly reducing launch costs. SpaceX’s Falcon 9 and Falcon Heavy rockets utilize advanced chemical propulsion systems that can be recovered and reused for multiple flights. Furthermore, NASA’s Artemis program leverages the Space Launch System (SLS) to support deep space missions, incorporating advanced chemical propulsion technologies to meet the demands of future exploration. Ongoing research into high-efficiency rocket engines, such as those powered by liquid oxygen and hydrogen, continues to drive progress in chemical propulsion, enhancing capabilities for missions beyond low Earth orbit.
Electric Propulsion
Electric propulsion offers a different approach to space travel by utilizing electrical energy to generate thrust. Unlike chemical propulsion, which relies on the combustion of propellants, electric propulsion systems use electrical power to accelerate ions or other particles to produce thrust. This method provides a more efficient means of propulsion for long-duration missions, as it can achieve higher specific impulses, which translates to better fuel efficiency. Electric propulsion is particularly advantageous for deep space missions where carrying large amounts of chemical propellants would be impractical.
Ion Thrusters and Their Efficiency
Ion thrusters are a type of electric propulsion system that uses electrical energy to ionize a propellant and accelerate the ions to produce thrust. These thrusters offer significant efficiency advantages over traditional chemical rockets, with a much higher specific impulse. Ion thrusters operate by generating a magnetic or electric field to accelerate ions expelled from the thruster, creating thrust. This allows spacecraft to achieve higher velocities with less propellant, making ion thrusters ideal for missions requiring prolonged propulsion, such as interplanetary travel or orbital adjustments. However, their thrust levels are lower compared to chemical rockets, making them suitable for missions where gradual acceleration is acceptable.
NASA’s Ongoing Electric Propulsion Projects
NASA is actively advancing electric propulsion technology through various projects and missions. The Dawn spacecraft, which used ion propulsion to explore the asteroid belt and dwarf planet Ceres, demonstrated the practical benefits of electric propulsion for deep space exploration. NASA’s NEXT (NASA’s Evolutionary Xenon Thruster) project is another significant effort aimed at developing high-performance ion propulsion systems for future missions. The agency is also exploring other electric propulsion technologies, such as Hall-effect thrusters, to enhance spacecraft maneuverability and efficiency. These ongoing projects are crucial for enabling long-duration missions and expanding the reach of human and robotic exploration in the solar system.
Nuclear Propulsion
Nuclear propulsion represents a promising frontier in space travel, utilizing nuclear reactions to generate thrust. This approach involves two primary concepts: nuclear thermal propulsion (NTP) and nuclear electric propulsion (NEP). In nuclear thermal propulsion, a nuclear reactor heats a propellant to high temperatures, which is then expelled to produce thrust. In contrast, nuclear electric propulsion involves using a nuclear reactor to generate electrical power, which is used to drive electric propulsion systems like ion thrusters. Both concepts aim to provide higher efficiency and greater thrust compared to chemical propulsion.
Concept and Potential Benefits
The concept of nuclear propulsion offers several potential benefits for deep space exploration. Nuclear thermal propulsion could significantly reduce travel times to distant planets by providing higher thrust compared to chemical rockets, making it ideal for crewed missions to Mars or beyond. Nuclear electric propulsion, on the other hand, could provide continuous, efficient thrust for long-duration missions, enabling spacecraft to travel further and faster. Both approaches have the potential to enhance mission capabilities, reduce travel times, and open new possibilities for exploration beyond the traditional limits of chemical propulsion. Additionally, nuclear propulsion could support the development of advanced space habitats and support missions to the outer planets and moons.
Research and Development Status
Research and development in nuclear propulsion are progressing, with several initiatives focused on overcoming technical and safety challenges. NASA and other space agencies, including the Department of Energy, are working on projects such as the Kilopower reactor, which aims to provide a compact and reliable power source for deep space missions. The development of nuclear thermal propulsion systems involves testing reactor designs, optimizing propellant performance, and ensuring safety protocols for handling nuclear materials. While significant progress has been made, including successful tests of key technologies, the practical implementation of nuclear propulsion for space missions is still in the development phase. Continued research is essential to address challenges related to reactor safety, radiation shielding, and integration with spacecraft systems.
Life Support Systems and Human Health
Advanced Life Support Systems
Advanced life support systems (ALSS) are critical for sustaining human life during extended space missions by providing essential life-supporting resources and managing waste. These systems are designed to replicate Earth-like conditions, ensuring that astronauts have access to breathable air, clean water, and adequate nutrition. The technology involves a combination of mechanical and biological processes to recycle air and water, manage waste products, and maintain a stable environment. ALSS must be highly reliable and efficient, as any failure can directly impact the health and safety of the crew. These systems are continuously evolving to enhance their efficiency and resilience, integrating new technologies to support long-duration missions beyond low Earth orbit.
Air and Water Recycling Technologies
Air and water recycling technologies are fundamental components of ALSS, enabling the closed-loop management of these critical resources. For air recycling, technologies such as the Carbon Dioxide Removal Assembly (CDRA) and the Oxygen Generation System (OGS) are used to remove CO2 from the cabin air and generate oxygen, ensuring a continuous supply of breathable air. Water recycling involves purifying and reusing wastewater through processes like filtration, distillation, and chemical treatments. The Advanced Water Recovery System (AWRS) is an example of a sophisticated water recycling system that can recover water from various sources, including humidity and urine. These technologies are essential for reducing the need for resupply missions and enhancing the sustainability of long-duration space missions.
Food Production and Storage Solutions
Food production and storage solutions are crucial for maintaining crew health and morale during extended space missions. Traditional methods of food storage, such as freeze-drying and dehydrating, are used to preserve food and minimize storage space. However, to support long-term missions, more advanced solutions are being developed, including space agriculture. Hydroponic and aeroponic systems allow for the cultivation of fresh produce in space, providing astronauts with nutritious food and psychological benefits from interacting with growing plants. Research is ongoing to optimize these systems and integrate them with existing life support technologies, ensuring a reliable and varied food supply for future deep space missions.
Radiation Protection
Understanding and managing space radiation is a critical aspect of ensuring astronaut safety during deep space missions. Space radiation, which includes cosmic rays and solar particle events, poses significant health risks such as increased cancer risk, radiation sickness, and damage to the central nervous system. Effective radiation protection strategies are necessary to mitigate these risks and safeguard crew members over long-duration missions.
Understanding Space Radiation
Space radiation originates from various sources, including galactic cosmic rays (GCRs) and solar energetic particles (SEPs). GCRs consist of high-energy protons and heavier ions that penetrate spacecraft and human tissue, while SEPs are associated with solar flares and coronal mass ejections. The space environment is devoid of Earth’s atmospheric and magnetic shielding, leading to higher exposure levels. Understanding the characteristics and behaviors of these radiation types is crucial for developing effective protective measures. Space agencies conduct extensive research to model radiation exposure and assess its potential health impacts on astronauts.
Shielding Techniques and Materials
Shielding techniques and materials are essential for protecting spacecraft and crew from the harmful effects of space radiation. Current strategies include using advanced materials and design approaches to attenuate radiation. High-density materials, such as polyethylene, are effective at stopping charged particles and reducing radiation dose. Innovations in shielding include hybrid materials that combine traditional shielding with active systems, such as magnetic fields or plasma shields, to provide additional protection. Research also focuses on incorporating radiation protection into spacecraft design and developing new materials with improved shielding properties. Effective shielding is vital for reducing astronauts’ radiation exposure and ensuring their health and safety during deep space missions.
Physical and Mental Health in Space
Maintaining physical and mental health is a crucial aspect of long-duration space missions. The microgravity environment of space can affect physical health in various ways, including muscle atrophy, bone density loss, and cardiovascular deconditioning. Additionally, the isolation and confinement of space can impact mental well-being, leading to issues such as stress, anxiety, and depression. Addressing these challenges involves implementing comprehensive health management strategies that include exercise, medical care, and psychological support.
Exercise and Fitness Regimens
Exercise and fitness regimens are essential for mitigating the effects of microgravity on the human body. In space, astronauts experience reduced gravity, which can lead to muscle atrophy and bone density loss. To counteract these effects, astronauts follow a rigorous exercise routine that includes cardiovascular, resistance, and flexibility training. The International Space Station (ISS) is equipped with specialized exercise equipment, such as treadmills, stationary bicycles, and resistance exercise devices, designed to function in microgravity. These regimens are tailored to maintain physical fitness, prevent health issues, and ensure astronauts are in optimal condition for mission tasks.
Psychological Support Strategies
Psychological support strategies are vital for maintaining mental health during extended space missions. The isolation, confinement, and distance from Earth can create psychological challenges for astronauts, including feelings of loneliness and stress. Space agencies implement various support systems to address these issues, such as regular communication with loved ones, virtual reality experiences, and recreational activities. Mental health professionals are also part of the crew support team, providing counseling and coping strategies. Crew members are trained to recognize and address mental health concerns, ensuring that any issues are managed proactively. These strategies aim to create a supportive environment and help astronauts maintain psychological well-being throughout their missions.
Navigation and Communication
Deep Space Network (DSN)
The Deep Space Network (DSN) is a critical component of space exploration infrastructure, providing essential communication capabilities for missions beyond Earth’s orbit. Operated by NASA, the DSN consists of a network of large radio antennas located at three sites around the globe: Goldstone in California, Madrid in Spain, and Canberra in Australia. This strategic positioning ensures continuous, global coverage and uninterrupted communication with spacecraft as they travel through deep space.
Structure and Capabilities
The DSN’s structure includes several high-gain parabolic antennas, each ranging from 34 to 70 meters in diameter. These antennas are equipped with advanced receivers and transmitters capable of handling high-frequency signals and large volumes of data. The DSN’s capabilities include tracking spacecraft, sending commands, and receiving scientific data from missions. The network supports a wide range of frequencies, including X-band and Ka-band, which are essential for high-data-rate communication. The DSN’s infrastructure also incorporates sophisticated signal processing and data handling systems to ensure accurate and reliable communication with distant spacecraft.
Role in Deep Space Missions
The DSN plays a pivotal role in deep space missions by providing continuous communication between mission control centers and spacecraft. For missions to the Moon, Mars, and beyond, the DSN enables real-time tracking and navigation, allows for the transmission of commands and instructions, and facilitates the receipt of scientific data and images. The network’s ability to maintain contact with spacecraft over vast distances is crucial for mission success, as it ensures that data can be collected and analyzed, and operational issues can be addressed promptly. The DSN’s support is vital for the execution of complex deep space missions, including those involving remote landers, rovers, and orbiters.
Autonomous Navigation
Autonomous navigation is an advanced technology that allows spacecraft to operate and navigate independently without constant input from Earth-based mission control. This capability is especially important for deep space missions, where communication delays can hinder real-time control. Autonomous navigation systems use onboard sensors, cameras, and algorithms to determine the spacecraft’s position and trajectory, make adjustments, and execute planned maneuvers. These systems are designed to handle unexpected situations, such as collisions with space debris, and ensure that the spacecraft remains on its intended path.
AI and Machine Learning Applications
Artificial intelligence (AI) and machine learning (ML) are increasingly being integrated into autonomous navigation systems to enhance their capabilities. AI algorithms can analyze vast amounts of data from spacecraft sensors, identify patterns, and make predictive decisions in real time. Machine learning models are trained on historical data and simulations to improve the accuracy and reliability of navigation systems. These technologies enable spacecraft to adapt to changing conditions, optimize their paths, and perform complex tasks with minimal human intervention. AI and ML applications are crucial for improving the efficiency and autonomy of deep space missions, allowing for more sophisticated and adaptive operations.
Real-Time Decision-Making Systems
Real-time decision-making systems are essential for managing spacecraft operations in deep space. These systems process data from various sensors and instruments, analyze it, and make decisions on-the-fly to address immediate challenges. For example, real-time systems can adjust spacecraft trajectories based on new information about potential hazards or mission objectives. These systems use advanced algorithms to prioritize tasks, execute commands, and ensure that the spacecraft operates efficiently and safely. Real-time decision-making is critical for handling the dynamic and unpredictable nature of deep space environments, where quick and accurate responses are necessary to achieve mission goals.
Laser Communication Systems
Laser communication systems represent a cutting-edge technology that offers several advantages over traditional radio wave communication. These systems use lasers to transmit data, providing a highly focused and high-bandwidth communication channel. The primary advantage of laser communication is its ability to deliver data at much higher rates compared to radio waves, allowing for the transmission of larger volumes of information and higher-resolution images. This is particularly valuable for deep space missions that generate substantial amounts of scientific data.
Advantages over Radio Waves
Laser communication systems offer several key advantages over radio waves, including higher data transfer rates, greater bandwidth, and improved signal clarity. Laser beams can carry more information per unit of time than radio waves, making them ideal for transmitting large datasets from distant spacecraft. Additionally, the narrow beam of a laser reduces signal loss and interference, resulting in more reliable communication. This capability is especially important for missions involving high-resolution imaging or complex scientific experiments, where the ability to transmit detailed data quickly and accurately is crucial.
Implementation and Testing
The implementation of laser communication systems involves the development and integration of advanced hardware and software components. This includes the design of high-precision laser transmitters and receivers, as well as the development of sophisticated signal processing algorithms. Testing is a critical phase of implementation, involving ground-based experiments and spaceborne trials to validate the system’s performance. For instance, NASA’s Laser Communications Relay Demonstration (LCRD) mission is designed to test laser communication technology and demonstrate its capabilities in space. These tests ensure that the system meets the necessary reliability and performance standards before being used in operational missions.
Extraterrestrial Resource Utilization (ISRU)
In-Situ Resource Utilization Basics
In-situ resource utilization (ISRU) refers to the process of using resources found at a mission site, such as the Moon or Mars, rather than relying on supplies transported from Earth. The concept of ISRU is crucial for sustainable space exploration, as it aims to minimize the need for resupply missions and reduce the cost and logistical complexity of space travel. By leveraging local resources, ISRU can provide essential materials and support the development of infrastructure directly on extraterrestrial surfaces. This approach not only enhances the feasibility of long-duration missions but also lays the groundwork for future human settlements beyond Earth.
Concept and Importance
The concept of ISRU involves extracting and utilizing resources available at a mission site to support human activities and infrastructure development. This includes producing essential materials such as water, oxygen, and construction materials from local resources. The importance of ISRU lies in its potential to significantly reduce the dependence on Earth for supplies, which is crucial for the sustainability of long-term space missions. By using local resources, space missions can achieve greater self-sufficiency, lower costs, and enhance the ability to establish permanent outposts or colonies. ISRU is a key enabler for exploring and settling other planets and celestial bodies, as it supports the creation of habitable environments and facilitates the construction of necessary infrastructure.
Moon and Mars ISRU Projects
ISRU projects for the Moon and Mars are actively being developed to support future exploration and habitation efforts. On the Moon, NASA’s Artemis program aims to establish a sustainable presence through ISRU technologies that will extract water from lunar regolith and ice deposits. This water can be used for life support, as well as to produce oxygen and hydrogen for rocket fuel. Similarly, Mars ISRU projects focus on extracting and utilizing local resources to support human missions. NASA’s Mars 2020 rover, Perseverance, includes experiments such as MOXIE (Mars Oxygen In-Situ Resource Utilization Experiment), which aims to demonstrate the production of oxygen from the Martian atmosphere. Both Moon and Mars ISRU projects are critical for enabling long-duration missions and the establishment of human presence on these celestial bodies.
Extracting Water and Oxygen
Extracting water and oxygen from local resources is a fundamental aspect of ISRU. On the Moon, water extraction involves mining lunar ice deposits or processing regolith to release trapped water. This water can be purified and split into hydrogen and oxygen through electrolysis, providing essential resources for life support and fuel production. On Mars, water extraction focuses on utilizing the planet’s ice deposits and atmospheric moisture. The production of oxygen from Martian CO2 is a key component of ISRU efforts, as demonstrated by the MOXIE experiment. Both processes are vital for supporting human life and enabling the production of propellants for return missions or further exploration.
Utilizing Local Materials for Construction
Utilizing local materials for construction is another critical aspect of ISRU that supports the development of infrastructure on extraterrestrial surfaces. On the Moon and Mars, local materials such as regolith and rocks can be used to create building materials like concrete or bricks. Techniques such as sintering, which involves heating materials to create solid structures, are explored for constructing habitats and other facilities. This approach reduces the need to transport construction materials from Earth, lowering costs and logistical challenges. Utilizing local resources also enables the creation of habitats and other structures that are better adapted to the local environment, enhancing the sustainability and resilience of space exploration efforts.
NASA’s Partnerships in ISRU
NASA’s approach to ISRU involves extensive partnerships with private companies, international space agencies, and research institutions. These collaborations are essential for advancing ISRU technologies and integrating them into mission plans. NASA works with companies specializing in space mining, manufacturing, and resource processing to develop and test ISRU systems. Partnerships also extend to international efforts, as global collaboration brings together diverse expertise and resources. Through these partnerships, NASA aims to accelerate the development of ISRU technologies, demonstrate their feasibility, and incorporate them into future space missions.
Collaborations with Private Companies
Collaborations with private companies play a significant role in advancing ISRU technologies. NASA partners with commercial entities to leverage their expertise in resource extraction, processing, and utilization. These partnerships enable the development of innovative solutions for extracting and using local resources on the Moon and Mars. For example, companies specializing in space mining are working with NASA to design systems for mining lunar regolith or Martian ice. Private companies also contribute to the development of technologies for in-situ manufacturing and construction. These collaborations are crucial for translating ISRU concepts into practical applications and supporting the long-term goals of space exploration.
International Cooperation
International cooperation is essential for advancing ISRU technologies and ensuring the success of space exploration missions. Collaborative efforts between space agencies, research institutions, and international organizations contribute to the development and testing of ISRU systems. For example, the European Space Agency (ESA) and other international partners collaborate with NASA on projects related to lunar and Martian exploration. Shared expertise, resources, and knowledge enhance the effectiveness of ISRU technologies and support the establishment of sustainable exploration and habitation strategies. International cooperation also fosters the development of global standards and practices for resource utilization, ensuring that space exploration efforts are coordinated and efficient.
Robotic Missions and Probes
Precursor Missions
Precursor missions are preliminary space missions designed to gather essential data and test technologies before more complex and ambitious missions are undertaken. These missions play a critical role in reducing risk and providing the necessary information to plan and execute future space exploration activities. By studying a target environment, precursor missions help identify suitable landing sites, evaluate resource availability, and test key systems and technologies. This foundational work is crucial for ensuring the success of subsequent missions, whether they involve human exploration, sample return, or in-situ resource utilization.
Role of Robotic Scouts
Robotic scouts play a vital role in precursor missions by exploring and analyzing environments that are otherwise inaccessible or too risky for human exploration. These robotic systems, including rovers and landers, are equipped with advanced sensors, cameras, and scientific instruments to conduct detailed surveys of planetary surfaces. Their primary functions include mapping terrain, assessing resource potential, and conducting preliminary scientific experiments. Robotic scouts provide valuable data that informs mission planning, enhances safety, and helps scientists understand the characteristics of celestial bodies. They are essential for paving the way for future missions and ensuring that exploratory efforts are well-informed and strategically targeted.
Key Missions and Their Findings
Several key precursor missions have significantly advanced our understanding of other planets and moons, providing critical insights for future exploration. The Mars rovers, such as Spirit, Opportunity, and Curiosity, have conducted extensive geological surveys, identified signs of past water activity, and assessed the habitability of Martian environments. The Lunar Reconnaissance Orbiter (LRO) has mapped the Moon’s surface in high detail, revealing potential landing sites and identifying water ice deposits. The Voyager missions provided the first close-up images of the outer planets and their moons, expanding our knowledge of the solar system. These missions have laid the groundwork for subsequent exploratory efforts by delivering crucial data and discoveries.
Sample Return Missions
Sample return missions involve collecting samples from other celestial bodies and bringing them back to Earth for detailed analysis. These missions provide invaluable scientific data that cannot be obtained through remote sensing alone. Techniques and technologies for sample return involve sophisticated collection methods, secure containment systems, and precise re-entry protocols. Notable sample return missions include the Apollo missions, which brought back lunar rocks and soil, and the Hayabusa2 and OSIRIS-REx missions, which collected samples from asteroids Ryugu and Bennu, respectively. These missions have expanded our understanding of the solar system’s origins and the composition of extraterrestrial materials.
Techniques and Technologies
Techniques and technologies used in sample return missions are highly advanced and specialized. For sample collection, methods such as drilling, scooping, and surface sampling are employed to gather representative materials. Containment systems ensure that samples are preserved and protected from contamination during transit. Re-entry technologies, including heat shields and precision landing systems, are designed to safely return samples to Earth. Recent advancements in robotics and automation have improved the efficiency and accuracy of these missions, allowing for more complex and ambitious sample return projects. These technologies are continuously evolving to support the growing demands of space exploration and scientific research.
Notable Missions and Discoveries
Notable missions and discoveries have significantly impacted our understanding of space and planetary science. The Apollo 11 mission, with its historic lunar landing, provided the first direct samples of lunar rock and soil, revolutionizing our knowledge of the Moon. The Mars Curiosity rover’s discovery of ancient stream beds and complex organic molecules indicated that Mars may have once had conditions suitable for life. The discovery of water ice on the Moon by the LCROSS mission highlighted the potential for using lunar resources to support future exploration. These missions have not only advanced scientific knowledge but also inspired further exploration and technological innovation.
Autonomous Robotics
Autonomous robotics are an integral part of modern space exploration, enabling spacecraft and landers to operate independently in challenging environments. These robots are equipped with advanced sensors, AI algorithms, and autonomous navigation systems that allow them to perform tasks without real-time human intervention. Autonomous robotics are capable of executing complex operations, such as terrain analysis, obstacle avoidance, and scientific experiments, in environments where direct control is impractical due to communication delays or remote locations. The development of autonomous robotics is crucial for enhancing mission efficiency and expanding the scope of exploration in deep space.
Rover and Drone Innovations
Innovations in rover and drone technology have significantly enhanced exploration capabilities on planetary surfaces. Modern rovers, such as NASA’s Perseverance and Curiosity, feature advanced mobility systems, high-resolution cameras, and sophisticated scientific instruments that enable detailed surface analysis and sample collection. These rovers are designed to navigate challenging terrain and perform autonomous operations to maximize exploration efficiency. Similarly, space drones, such as the Ingenuity helicopter on Mars, represent a new frontier in aerial exploration, providing a unique perspective and enabling reconnaissance of hard-to-reach areas. These innovations improve our ability to explore and understand extraterrestrial environments, paving the way for future missions.
Future Robotic Mission Plans
Future robotic mission plans involve developing and deploying increasingly advanced systems to explore the Moon, Mars, and beyond. Upcoming missions will focus on a range of objectives, including sample collection, resource utilization, and scientific discovery. For example, NASA’s Lunar Gateway program aims to deploy robotic systems to support human missions and conduct scientific research in lunar orbit. Mars missions will continue to explore the planet’s surface and subsurface, with upcoming rovers and landers designed to search for signs of past life and assess potential habitability. Robotic missions to asteroids, outer planets, and their moons are also in the planning stages, with goals including resource exploration, planetary defense, and understanding the formation of the solar system. These future missions will leverage advancements in robotics, AI, and materials science to achieve new milestones in space exploration.
Training and Preparing Astronauts
Simulation and Training Facilities
Simulation and training facilities are essential for preparing astronauts and mission control teams for the complexities of space exploration. These facilities provide controlled environments where personnel can practice and refine their skills, experience realistic space mission scenarios, and troubleshoot potential issues before actual missions. They include a range of systems and technologies designed to replicate various aspects of space missions, from spacecraft operations to extravehicular activities (EVAs) and mission control procedures. By immersing trainees in simulations that closely mimic real mission conditions, these facilities help ensure that both astronauts and ground teams are well-prepared for the challenges of space exploration.
Virtual Reality and Simulators
Virtual reality (VR) and advanced simulators are pivotal tools in astronaut training and mission preparation. VR systems create immersive, interactive environments that allow trainees to experience and interact with spacecraft systems, extraterrestrial surfaces, and mission scenarios in a highly realistic manner. These simulations help astronauts practice operations, familiarize themselves with spacecraft layouts, and rehearse emergency procedures. Simulators, which can range from cockpit mock-ups to full-motion platforms, replicate the physical sensations of space travel, such as acceleration and weightlessness. These tools enhance training effectiveness by providing hands-on experience and enabling trainees to address complex situations in a safe, controlled setting.
Neutral Buoyancy Lab
The Neutral Buoyancy Lab (NBL) is a specialized facility used to simulate the microgravity environment of space. It features a large swimming pool where astronauts and equipment can be submerged to replicate the conditions of zero-gravity. This environment allows for the practice of extravehicular activities (EVAs), testing of spacecraft components, and training for maneuvers that would be performed in space. The NBL is instrumental in helping astronauts become accustomed to the movements and challenges of operating in a weightless environment, ensuring that they are well-prepared for the realities of spacewalks and other mission tasks.
Long-Duration Mission Training
Long-duration mission training prepares astronauts for extended stays in space, where they will face unique challenges related to isolation, confinement, and the prolonged absence of Earth’s environmental conditions. This training includes simulations that mimic the psychological and physical stresses of long-term missions, such as living in confined spaces, managing sleep schedules, and maintaining physical fitness. Training programs also address the management of daily routines, team dynamics, and coping strategies for dealing with the monotony and isolation of extended missions. The goal is to ensure that astronauts are equipped to handle the demands of long-duration missions and maintain their health and well-being throughout the mission.
Mars Analog Missions
Mars analog missions are simulated missions conducted on Earth to replicate the conditions of a Mars exploration mission. These analogs provide valuable insights into the challenges and requirements of living and working on Mars. They typically involve setting up a habitat in a remote or extreme environment, such as a desert or volcanic region, where participants live and work under conditions that simulate those on Mars. Analog missions allow scientists and engineers to test equipment, study human factors, and develop operational procedures in a setting that closely resembles the Martian environment. These missions are crucial for refining mission plans, understanding potential issues, and preparing for the realities of Mars exploration.
Psychological and Physical Preparedness
Psychological and physical preparedness are critical components of astronaut training, ensuring that individuals are equipped to handle the stresses of space missions. Psychological preparedness involves training in stress management, teamwork, and coping strategies for isolation and confinement. Techniques such as counseling, behavioral therapy, and stress management exercises are employed to support mental health. Physical preparedness includes maintaining physical fitness through exercise regimens tailored to the microgravity environment, as well as training for the physical demands of space operations. Both aspects of preparedness are essential for ensuring that astronauts can perform effectively and maintain their health during missions.
International Collaboration in Training
International collaboration in training enhances the effectiveness and scope of astronaut preparation by bringing together diverse expertise and resources from around the world. Space agencies from different countries often work together on training programs, sharing best practices, facilities, and technologies. This collaboration allows for a more comprehensive approach to training, incorporating a range of perspectives and experiences. Joint training efforts also facilitate the development of standardized procedures and protocols, improving coordination and interoperability among international space missions. By working together, space agencies can provide more robust and diverse training opportunities for astronauts.
Joint Training Programs
Joint training programs involve collaboration between multiple space agencies or organizations to develop and deliver training for astronauts and mission personnel. These programs may include shared simulations, cross-training exercises, and joint research initiatives. The goal is to leverage the strengths and expertise of each participating organization to create more effective and comprehensive training experiences. Joint training programs also foster greater international cooperation and understanding, which is essential for successful collaborative space missions. Examples of joint training programs include the International Space Station (ISS) training efforts, where astronauts from different countries train together to prepare for their time aboard the station.
Sharing Knowledge and Resources
Sharing knowledge and resources among space agencies and organizations is crucial for advancing space exploration and improving training programs. This collaboration involves exchanging information on best practices, research findings, and technological developments. By sharing resources such as training facilities, simulation tools, and expert knowledge, space agencies can enhance their training programs and reduce costs. Collaborative efforts also enable the development of standardized protocols and systems, improving the efficiency and effectiveness of space missions. Sharing knowledge and resources fosters a more integrated and cooperative approach to space exploration, benefiting all participants and advancing the goals of human spaceflight.
Challenges and Solutions
Psychological and Social Challenges
Psychological and social challenges are significant concerns for astronauts during space missions, particularly those involving long-duration stays. The isolation and confinement of space environments can lead to feelings of loneliness, stress, and anxiety. The lack of physical contact with loved ones and the separation from familiar social structures can exacerbate these feelings. Additionally, the small, confined spaces and the close quarters with fellow crew members can lead to interpersonal conflicts and stress. Addressing these challenges requires comprehensive support systems, including psychological counseling, stress management techniques, and strategies for maintaining social connections with family and friends. Effective management of these issues is essential for ensuring crew well-being and mission success.
Coping with Isolation and Confined Spaces
Coping with isolation and confined spaces is crucial for maintaining mental health and operational efficiency during space missions. Astronauts must adapt to living in small, enclosed habitats where personal space is limited and social interactions are constant. To manage these conditions, astronauts engage in routine activities, exercise, and hobbies to maintain psychological well-being. Structured schedules and regular communication with Earth help reduce feelings of isolation. Training in conflict resolution and stress management is also vital, as it prepares astronauts to handle interpersonal issues and maintain a positive group dynamic. Ensuring that astronauts have coping strategies and support systems in place is essential for their overall health and mission performance.
Team Dynamics and Conflict Resolution
Team dynamics and conflict resolution are critical aspects of space missions, where crew members must work closely together under high-stress conditions. Effective teamwork is essential for mission success, as astronauts must coordinate their efforts, share responsibilities, and support each other. Training programs focus on developing strong interpersonal skills, communication strategies, and conflict resolution techniques. Crew members learn to navigate interpersonal conflicts, manage stress, and collaborate effectively despite the challenging environment. Regular team-building exercises and psychological support help maintain a positive group dynamic and ensure that crew members can work together harmoniously throughout the mission.
Technical and Engineering Challenges
Technical and engineering challenges are inherent in space missions, where complex systems must operate reliably in harsh environments. Key challenges include designing and maintaining spacecraft systems, ensuring their functionality in microgravity, and addressing failures or malfunctions. Engineers must develop robust systems for life support, power generation, and communications, as well as ensure that these systems can withstand the extremes of space. Testing and redundancy are critical to minimize the risk of system failures. Continuous monitoring and maintenance are required to address any issues that arise. Overcoming these technical challenges is essential for the safety and success of space missions.
Long-Distance Communication Delays
Long-distance communication delays present significant challenges for space missions, particularly those involving distant destinations like Mars. The time it takes for signals to travel between Earth and the spacecraft can range from minutes to hours, depending on the distance. This delay affects real-time communication and requires astronauts to make autonomous decisions and execute tasks independently. Mission control teams must plan and coordinate activities with these delays in mind, using pre-programmed instructions and automated systems to manage operations. Effective strategies for handling communication delays include robust onboard systems for data processing and decision-making, as well as carefully planned communication schedules to ensure that critical information is exchanged.
Repair and Maintenance in Space
Repair and maintenance in space are crucial for ensuring the continued functionality of spacecraft and equipment. Given the absence of readily available repair resources and the complexity of space systems, astronauts must be trained to perform a wide range of maintenance tasks. This includes fixing mechanical and electrical systems, replacing components, and addressing system malfunctions. Spacecraft are equipped with toolkits and spare parts, and astronauts receive detailed training on how to use these tools and perform repairs. The ability to conduct repairs and maintenance in space is essential for addressing issues that arise during missions and ensuring that systems remain operational for the duration of the mission.
Environmental and Sustainability Challenges
Environmental and sustainability challenges are important considerations for space missions, particularly those involving long-duration stays or the establishment of permanent outposts. Managing the space environment involves addressing issues related to resource utilization, waste management, and the impact on the space environment itself. Ensuring that missions are sustainable requires developing practices for efficient resource use, minimizing waste, and mitigating environmental impacts. This includes strategies for recycling air and water, managing waste products, and using local resources to reduce the need for resupply from Earth. Addressing these challenges is critical for the long-term sustainability of space exploration and the development of space habitats.
Managing Waste in Space
Managing waste in space involves developing systems and procedures for handling various types of waste produced during missions. This includes biological waste, packaging materials, and other debris. Waste management systems must be designed to safely contain and process waste materials, preventing contamination of the spacecraft environment and minimizing the impact on mission operations. Technologies for waste processing and recycling are employed to convert waste into useful resources, such as water or energy. Effective waste management is essential for maintaining a clean and safe environment on spacecraft and ensuring that resources are used efficiently.
Sustainable Practices for Long Missions
Sustainable practices for long missions focus on minimizing the environmental impact of space exploration and ensuring that resources are used efficiently. This includes implementing strategies for recycling air, water, and waste, as well as utilizing in-situ resources to reduce the reliance on supplies transported from Earth. Sustainable practices also involve the development of energy-efficient technologies and systems that reduce the consumption of resources and minimize waste. By adopting these practices, space missions can enhance their self-sufficiency, reduce operational costs, and support the long-term goal of establishing permanent human presence in space.
The Future of Deep Space Travel
NASA’s Artemis Program
NASA’s Artemis program is a pioneering initiative aimed at returning humans to the Moon and establishing a sustainable presence there, with the broader goal of enabling future missions to Mars and beyond. Named after Artemis, the twin sister of Apollo, the program seeks to build on the legacy of the Apollo missions by addressing new challenges and leveraging advancements in technology. Artemis aims to land the first woman and the next man on the lunar surface, particularly focusing on the lunar South Pole due to its potential water ice deposits. The program is designed to lay the groundwork for long-term human exploration of the solar system.
Goals and Timeline
The Artemis program’s goals include establishing a sustainable lunar presence, advancing scientific knowledge, and testing new technologies for future deep space missions. The timeline is structured around several key milestones: Artemis I, an uncrewed test flight of the Space Launch System (SLS) and Orion spacecraft, is scheduled for 2024. Artemis II will be the first crewed mission of the Orion spacecraft, orbiting the Moon and returning to Earth, tentatively set for 2025. Artemis III aims to land astronauts on the lunar surface, with a focus on the South Pole, projected for 2026. Following these missions, NASA plans to establish the Lunar Gateway and build a Lunar Base to support continuous exploration and research.
Artemis Missions Overview
The Artemis missions are designed to achieve several key objectives, including human landings on the Moon, particularly the South Pole, to conduct scientific research and test new technologies. The establishment of the Lunar Gateway will provide a space station in lunar orbit, serving as a staging point for surface missions and a testbed for deep space technologies. The Lunar Base will support extended human exploration and research. The missions also aim to advance scientific knowledge about the Moon and demonstrate the feasibility of technologies needed for future Mars exploration.
Mars and Beyond
Long-Term Goals for Human Exploration
NASA’s long-term goals for human exploration extend to Mars and beyond, with Artemis serving as a foundation. The objectives include developing and testing technologies for Mars landing, surface operations, and sustainable living. This involves creating habitats that support human life for extended periods and exploring and utilizing resources on Mars and the Moon. Advancements in propulsion systems and space infrastructure are also targeted to enable long-duration missions to Mars and other destinations within the solar system.
Planned Missions and Milestones
Several key missions and milestones will support the goal of exploring Mars and beyond. The Lunar Gateway, set to be operational by the late 2020s, will provide a platform for deep space missions and serve as a staging point for Mars exploration. The development of the lunar base will support long-term missions and test critical technologies. Planning and technology development for crewed Mars missions are expected to begin in the 2030s, with a focus on advancing propulsion systems, radiation protection, and life support technologies.
Vision for the Next Decades
Potential Technologies on the Horizon
Looking ahead, several technologies will be crucial for advancing human space exploration. Nuclear propulsion systems, including nuclear thermal and nuclear electric propulsion, are being developed to reduce travel time to Mars. Advanced life support systems for recycling air, water, and waste, and producing food in space, are also under development. Innovations in habitat technologies will create expandable and sustainable living environments for use on the Moon and Mars. Enhanced robotic systems will support exploration, construction, and resource extraction on extraterrestrial surfaces.
Future Collaborations and Partnerships
Future space exploration will benefit significantly from collaborations and partnerships. International partnerships will involve working with space agencies and organizations worldwide to share expertise and resources. Private sector engagement will help develop new technologies, commercialize space activities, and support infrastructure. Collaborations with academic and research institutions will advance scientific knowledge, develop innovative technologies, and conduct critical research. These partnerships will accelerate progress, reduce costs, and expand the scope of human space exploration.
Conclusion
Recap of NASA’s Contributions to Deep Space Travel
NASA has been at the forefront of deep space exploration, making significant contributions through a series of groundbreaking missions and technological advancements. From the early Apollo missions that landed humans on the Moon to the development of the Artemis program, NASA has consistently pushed the boundaries of space exploration. The agency’s work on spacecraft like the Orion and the Space Launch System (SLS) has been crucial in preparing for future deep space missions. Innovations in propulsion systems, such as chemical, electric, and nuclear propulsion, have advanced the capabilities needed for long-duration space travel. NASA’s development of advanced life support systems and radiation protection technologies has laid the groundwork for human missions beyond low Earth orbit. The agency’s achievements in establishing the Deep Space Network (DSN) and advancing autonomous navigation further demonstrate its leadership in exploring the far reaches of our solar system. Through these efforts, NASA has not only expanded our understanding of space but has also set the stage for future exploration of Mars and beyond.
The Promise and Potential of Future Exploration
The future of space exploration holds tremendous promise and potential, driven by ongoing advancements and ambitious goals. As NASA continues to develop and refine technologies, the possibilities for deep space travel and exploration expand. The Artemis program, with its goal of returning humans to the Moon and establishing a sustainable presence, is a stepping stone to more distant missions. Future exploration efforts aim to build on these achievements, with plans for crewed missions to Mars and the establishment of long-term human habitats on the Red Planet. Emerging technologies, such as advanced propulsion systems and in-situ resource utilization, are expected to revolutionize space travel, making it more efficient and sustainable. The potential for discovering new scientific knowledge, harnessing extraterrestrial resources, and expanding human presence across the solar system presents exciting opportunities. Collaboration with international partners and private industry will further enhance the capabilities and scope of exploration, driving humanity toward a new era of discovery and innovation in space.
FAQs
What is NASA’s Role in Deep Space Exploration?
NASA plays a pivotal role in deep space exploration as the leading agency responsible for advancing our capabilities to explore beyond Earth’s orbit. The agency drives innovation through its development of spacecraft, launch systems, and scientific instruments designed for deep space missions. NASA’s efforts include the design and implementation of groundbreaking missions, such as the Artemis program aimed at returning humans to the Moon and establishing a base for future Mars exploration. The agency also conducts fundamental research on space environments and develops technologies for life support, propulsion, and communication needed for long-duration missions. By leading the development of key technologies and conducting high-profile missions, NASA sets the agenda for deep space exploration and provides the foundation for future discoveries.
How Does NASA Ensure the Safety of Astronauts on Long Missions?
NASA ensures the safety of astronauts on long missions through a comprehensive approach that includes rigorous training, advanced technology, and robust support systems. Astronauts undergo extensive training in simulation facilities to prepare for various scenarios they may encounter in space. This training covers technical skills, emergency procedures, and psychological resilience. NASA also invests in advanced life support systems that recycle air, water, and waste to create a sustainable living environment. Radiation protection measures are implemented to shield astronauts from harmful cosmic rays and solar radiation. Additionally, continuous health monitoring and support systems are in place to address any medical issues that may arise during missions. These combined efforts help mitigate risks and ensure the well-being of astronauts during extended space flights.
What Technological Advancements are Critical for Deep Space Travel?
Several technological advancements are critical for deep space travel, each addressing specific challenges associated with long-duration missions. Advanced propulsion systems, such as nuclear thermal and electric propulsion, are crucial for reducing travel time and increasing efficiency in deep space. Life support technologies that enable recycling of air, water, and waste are essential for sustaining human life in space. Radiation shielding materials and techniques are needed to protect astronauts from cosmic and solar radiation. Additionally, improvements in communication technologies, including high-bandwidth systems and autonomous navigation, are vital for maintaining contact with mission control and ensuring safe navigation. Developing these technologies is key to overcoming the challenges of deep space exploration and enabling future missions to distant destinations.
How Does NASA Collaborate with Other Space Agencies and Private Companies?
NASA collaborates with other space agencies and private companies through a variety of partnerships and agreements designed to leverage shared expertise and resources. International collaborations involve working with space agencies such as the European Space Agency (ESA), Roscosmos, and the Japanese Aerospace Exploration Agency (JAXA) on joint missions, research, and technology development. These partnerships enhance capabilities and facilitate the sharing of knowledge and resources. NASA also engages with private companies through programs like the Commercial Crew Program and Public-Private Partnerships (PPPs) to develop new technologies, services, and infrastructure. These collaborations help drive innovation, reduce costs, and accelerate progress in space exploration by combining the strengths of both public and private sectors.
What Are the Biggest Challenges Faced in Preparing for Deep Space Travel?
Preparing for deep space travel presents several significant challenges. One of the foremost challenges is developing reliable and efficient propulsion systems capable of carrying spacecraft to distant destinations and returning safely. Another major challenge is ensuring the safety and well-being of astronauts during long-duration missions, which involves addressing issues related to isolation, confinement, and health. Life support systems must be designed to sustain human life by recycling resources and managing waste effectively. Additionally, dealing with the harsh environment of space, including radiation exposure and microgravity effects, requires innovative solutions. Finally, logistical challenges, such as ensuring continuous communication over vast distances and managing resources and supplies, are crucial for mission success. Addressing these challenges requires ongoing research, technological advancements, and comprehensive planning.