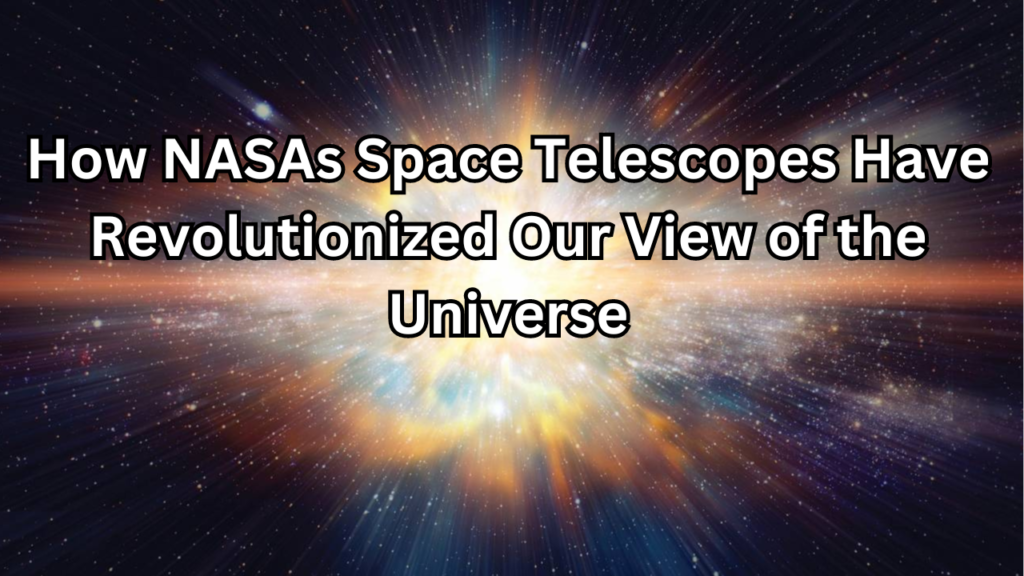
Table of Contents
Introduction
NASAs space telescopes have transformed our understanding of the universe in ways previously unimaginable. From unveiling the hidden structure of our cosmos to discovering new planets beyond our solar system, these powerful instruments have provided insights that have reshaped modern astronomy. This article explores the profound impact of NASA’s space telescopes, highlighting their contributions to astronomy and their importance in contemporary scientific research.
Overview of NASA’s Contribution to Astronomy
Since its inception, NASA has played a pivotal role in advancing astronomical research. The agency’s commitment to exploring space has led to the development and deployment of numerous space telescopes, each designed to observe the universe in different wavelengths of light. These telescopes have allowed scientists to peer deeper into space and time, revealing phenomena that are invisible to ground-based observatories due to the Earth’s atmosphere.
NASA’s contributions to astronomy are vast and varied. They include the launch of the Hubble Space Telescope, which has provided some of the most detailed images of the cosmos ever captured, and the Kepler Space Telescope, which has identified thousands of potential exoplanets. Other significant missions, such as the Chandra X-ray Observatory and the Spitzer Space Telescope, have expanded our understanding of high-energy phenomena and the infrared universe, respectively. Through these efforts, NASA has not only expanded our knowledge of the universe but also inspired generations of scientists and enthusiasts to look to the stars.
Importance of Space Telescopes in Modern Astronomy
Space telescopes are crucial to modern astronomy for several reasons. First and foremost, they overcome the limitations imposed by Earth’s atmosphere. Ground-based telescopes must contend with atmospheric distortion, light pollution, and weather conditions, all of which can obscure and degrade astronomical observations. Space telescopes, positioned above the Earth’s atmosphere, can capture clearer and more detailed images of celestial objects.
Moreover, space telescopes are capable of observing the universe in a wide range of wavelengths, including ultraviolet, X-ray, and infrared, which are largely absorbed by the Earth’s atmosphere and therefore inaccessible to ground-based instruments. This ability allows astronomers to study phenomena such as black holes, star formation, and distant galaxies in unprecedented detail.
Another significant advantage of space telescopes is their continuous operation. Unlike ground-based telescopes, which can only observe the night sky and are subject to interruptions, space telescopes can operate around the clock. This continuous observation capability is particularly important for monitoring transient events, such as supernovae and gamma-ray bursts, as well as for conducting long-term studies of variable stars and other time-sensitive phenomena.
History of NASA’s Space Telescopes
Early Efforts and Ground-Based Telescopes
Before the advent of space telescopes, astronomers relied exclusively on ground-based telescopes to study the universe. These early telescopes, from Galileo’s modest instrument to the giant observatories of the 20th century, were pivotal in advancing our understanding of the cosmos. Ground-based telescopes, situated on mountain tops and remote locations, aimed to minimize atmospheric interference and maximize observational clarity.
Pioneers like Galileo Galilei and Johannes Kepler laid the foundation for modern astronomy with their telescopic observations, revealing moons around Jupiter, the phases of Venus, and the detailed surface of the Moon. Over the centuries, technological advancements led to the construction of larger and more sophisticated telescopes. The 100-inch Hooker Telescope at Mount Wilson Observatory and the 200-inch Hale Telescope at Palomar Observatory, for example, enabled astronomers to explore distant galaxies and nebulae, deepening our understanding of the universe’s structure.
Despite their achievements, ground-based telescopes faced significant limitations. The Earth’s atmosphere causes turbulence, leading to blurry images—a phenomenon known as “atmospheric seeing.” Light pollution from human activity further compromised the visibility of faint celestial objects. Additionally, certain wavelengths of electromagnetic radiation, such as ultraviolet and X-rays, are absorbed by the atmosphere and cannot be observed from the ground. These challenges necessitated the development of alternative methods to observe the cosmos, leading to the birth of space telescopes.
The Birth of Space Telescopes
The concept of space telescopes emerged from the need to overcome the limitations of ground-based observations. The idea was to place telescopes above the Earth’s atmosphere, allowing them to capture unobstructed and clearer images of the universe. This vision became a reality with the launch of the first space-based observatories in the mid-20th century.
The Orbiting Astronomical Observatory (OAO) program, initiated by NASA in the 1960s, marked the beginning of space-based astronomy. OAO-2, launched in 1968, was the first successful space telescope, providing valuable ultraviolet observations of stars and galaxies. These early missions demonstrated the feasibility and scientific value of space telescopes, setting the stage for more advanced instruments.
The Hubble Space Telescope (HST), launched in 1990, represented a significant leap forward in space telescope technology. Hubble’s position above the Earth’s atmosphere allowed it to capture images with unprecedented clarity and detail. Despite initial challenges, such as a flawed primary mirror that required a subsequent servicing mission, Hubble became one of the most successful and influential space telescopes in history. Its observations have led to numerous groundbreaking discoveries, including the accelerated expansion of the universe and detailed studies of distant galaxies and nebulae.
Following Hubble’s success, NASA launched several other specialized space telescopes, each designed to observe different wavelengths of light and investigate specific astronomical phenomena. The Chandra X-ray Observatory, launched in 1999, focused on high-energy X-ray emissions from hot gas, black holes, and neutron stars. The Spitzer Space Telescope, launched in 2003, specialized in infrared observations, revealing the hidden structure of dust clouds and star-forming regions.
These pioneering missions demonstrated the immense potential of space telescopes and paved the way for future advancements. The continuous development and deployment of space telescopes have revolutionized our understanding of the universe, providing insights into the origins, structure, and evolution of celestial objects and phenomena. As technology advances, new and more sophisticated space telescopes continue to push the boundaries of astronomical research, promising even greater discoveries in the years to come.
The Pioneers: Early NASA Space Telescopes
Orbiting Astronomical Observatory (OAO)
The Orbiting Astronomical Observatory (OAO) program was a groundbreaking initiative by NASA to take astronomical observations beyond the confines of Earth’s atmosphere. Launched in the 1960s, the OAO series marked the beginning of space-based astronomy, providing astronomers with an unprecedented view of the universe. The first satellite, OAO-1, launched in 1966, suffered a power failure shortly after reaching orbit, but its successor, OAO-2, launched in 1968, achieved significant success. OAO-2 carried two main instruments: the Wisconsin Experiment Package (WEP), designed to study ultraviolet (UV) spectra of stars, and the Celescope, which conducted UV photometry. These instruments allowed astronomers to study celestial objects in the ultraviolet spectrum for the first time, revealing details about stars and galaxies that were invisible from ground-based telescopes.
OAO-2’s observations contributed to a better understanding of the composition and behavior of stars, particularly hot, young stars that emit significant amounts of ultraviolet radiation. The mission also provided data on interstellar dust and gas, enhancing knowledge of the medium through which light from distant stars travels. Following the success of OAO-2, NASA launched OAO-B in 1970, but it failed during launch. However, the final mission of the program, OAO-3, also known as Copernicus, launched in 1972, was a resounding success. OAO-3 carried an ultraviolet telescope and a far-ultraviolet spectrometer, focusing on studying the interstellar medium, the structure of galaxies, and the characteristics of stars. Copernicus made numerous significant contributions to astronomy, including detailed observations of the ultraviolet spectra of stars and the detection of interstellar molecules. The OAO program was instrumental in demonstrating the feasibility and value of space-based astronomical observations, paving the way for future missions like the Hubble Space Telescope and other advanced observatories.
International Ultraviolet Explorer (IUE)
The International Ultraviolet Explorer (IUE) was a collaborative space mission involving NASA, the European Space Agency (ESA), and the United Kingdom’s Science and Engineering Research Council (SERC). Launched on January 26, 1978, IUE was designed to study the ultraviolet universe, focusing on a wide range of astronomical phenomena. Featuring a 45-centimeter primary mirror and three spectrographs, IUE could observe ultraviolet light from 115 to 320 nanometers. Unlike previous space telescopes, IUE was designed for long-term operation and was controlled in real-time by astronomers on Earth. This capability enabled astronomers to adjust observations based on immediate data, making IUE an incredibly flexible and responsive instrument.
Over its 18-year mission, IUE made numerous significant contributions to various fields of astronomy. It provided detailed spectra of different types of stars, from young, hot stars to older, cooler stars, improving models of stellar atmospheres and the life cycles of stars. IUE’s ability to monitor supernovae and novae in the ultraviolet spectrum provided insights into the mechanisms of these explosive events and the nature of the interstellar medium they interact with. The telescope’s observations of galaxies contributed to understanding their star formation rates, chemical compositions, and evolutionary processes. Additionally, IUE’s data on active galactic nuclei (AGN) helped elucidate the nature of these powerful sources of radiation. Observations of comets revealed details about their composition and the processes occurring in their comae and tails, enhancing understanding of these primitive solar system objects.
The International Ultraviolet Explorer was the longest-running astronomical satellite of its time, operating until 1996. Its vast archive of ultraviolet spectra remains a valuable resource for astronomers, and its success demonstrated the effectiveness of international collaboration in space science. IUE’s legacy is seen in the subsequent generation of space telescopes, including the Hubble Space Telescope, which continues to build on the foundation laid by IUE. The mission’s extensive contributions to our understanding of the ultraviolet universe have left a lasting impact on the field of astronomy, influencing both scientific research and the development of future space observatories.
Hubble Space Telescope: A Giant Leap
Development and Launch of Hubble
The Hubble Space Telescope (HST) stands as one of NASA’s most ambitious and successful projects, revolutionizing our understanding of the cosmos. The development of Hubble began in the 1970s, following the success of earlier space observatories. NASA partnered with the European Space Agency (ESA) to create a versatile and powerful space telescope capable of observing the universe in visible, ultraviolet, and near-infrared light. The telescope was named after the astronomer Edwin Hubble, whose observations in the 1920s revealed the expansion of the universe.
The Hubble Space Telescope was designed to be serviceable, allowing astronauts to perform maintenance and upgrades. Its development faced numerous technical, financial, and logistical challenges, but after years of rigorous testing and refinement, Hubble was finally ready for launch. On April 24, 1990, the Space Shuttle Discovery carried Hubble into orbit, placing it approximately 547 kilometers (340 miles) above Earth. This vantage point allowed Hubble to bypass the distortions caused by Earth’s atmosphere, providing unprecedented clarity in its observations of the universe.
Technical Challenges and Solutions
Shortly after its launch, the Hubble Space Telescope encountered a major setback. Scientists discovered that the primary mirror, the heart of the telescope, had been ground to the wrong shape due to a flaw in the testing equipment. This spherical aberration caused images to be blurred, significantly compromising Hubble’s capabilities. The revelation was a significant blow to NASA and the astronomical community, as expectations for Hubble’s performance were extraordinarily high.
In response to this crisis, NASA devised a plan for an ambitious repair mission. In December 1993, astronauts aboard the Space Shuttle Endeavour conducted the first servicing mission (STS-61). They installed the Corrective Optics Space Telescope Axial Replacement (COSTAR), a set of small mirrors designed to correct the optical error, and replaced several of Hubble’s instruments with upgraded versions. This mission was a resounding success, effectively restoring Hubble’s imaging capabilities and proving that on-orbit servicing was feasible. Subsequent servicing missions in 1997, 1999, 2002, and 2009 further enhanced Hubble’s instrumentation, extending its operational life and scientific capabilities.
Major Discoveries by Hubble
Since its repair and subsequent upgrades, the Hubble Space Telescope has made numerous groundbreaking discoveries, transforming our understanding of the universe. One of its most famous contributions is the Hubble Deep Field images, which captured thousands of previously unseen galaxies in a small region of the sky, offering a glimpse into the early universe and providing evidence for the vast scale of the cosmos.
Hubble also played a crucial role in determining the rate of expansion of the universe, refining the value of the Hubble constant and supporting the theory of dark energy. Its observations of distant supernovae provided strong evidence that the expansion of the universe is accelerating, a discovery that earned the 2011 Nobel Prize in Physics for the scientists involved.
In addition to these cosmological achievements, Hubble has made significant contributions to our understanding of planetary science. It has observed the weather patterns and atmospheric compositions of planets in our solar system, discovered moons around Pluto, and provided detailed images of the outer planets and their rings. Hubble’s ability to detect and study exoplanets has also opened new avenues in the search for potentially habitable worlds beyond our solar system.
The Hubble Space Telescope’s legacy is one of profound scientific discovery and inspiration. It has not only expanded our knowledge of the universe but also captured the public’s imagination with its stunning images, highlighting the beauty and complexity of the cosmos. As Hubble continues to operate, it remains a vital tool for astronomers, continuing to push the boundaries of our understanding of the universe.
Chandra X-ray Observatory: Seeing the Invisible
Introduction to Chandra
The Chandra X-ray Observatory is one of NASA’s premier space telescopes, dedicated to observing the universe in X-rays, a type of high-energy radiation that is absorbed by Earth’s atmosphere and thus cannot be detected from the ground. Launched on July 23, 1999, aboard the Space Shuttle Columbia, Chandra was named in honor of the Indian-American astrophysicist Subrahmanyan Chandrasekhar, whose work on stellar evolution and black holes profoundly influenced modern astrophysics. Designed to explore some of the most energetic and extreme phenomena in the universe, Chandra has provided unparalleled insights into the high-energy universe, from the remnants of exploded stars to the powerful jets emitted by black holes.
Technological Advancements and Design
Chandra’s design incorporates several cutting-edge technologies to maximize its ability to capture and analyze X-rays. Unlike optical telescopes, which use mirrors to gather light, Chandra employs a unique system of nested, concentric mirrors made of highly reflective materials. These mirrors are specially designed to focus X-rays, which have much higher energies than visible light and thus require a different optical approach. The telescope’s mirrors are coated with a layer of a specialized material called a grazing incidence coating, which allows X-rays to be reflected rather than absorbed.
The observatory also features an array of sophisticated detectors, including the Advanced CCD Imaging Spectrometer (ACIS) and the High Resolution Camera (HRC). ACIS captures high-resolution images and spectra of X-ray sources, while HRC provides extremely detailed images with very fine spatial resolution. Chandra’s orbit around Earth is highly elliptical, allowing it to stay above the radiation belts and provide continuous observations of celestial X-ray sources.
The spacecraft itself is equipped with a high-precision attitude control system to maintain its orientation and stability. This system ensures that Chandra can focus on specific celestial objects for extended periods, providing high-quality data on X-ray emissions from distant and faint sources.
Significant Contributions to X-ray Astronomy
Since its launch, the Chandra X-ray Observatory has made numerous groundbreaking contributions to the field of X-ray astronomy. One of its major achievements is the detailed study of supernova remnants. Chandra’s observations have provided insights into the mechanisms behind these explosive events and the formation of neutron stars and black holes. By analyzing the X-ray emissions from these remnants, scientists have been able to trace the distribution of heavy elements produced in supernova explosions, contributing to our understanding of the lifecycle of stars and the chemical enrichment of the universe.
Chandra has also made significant advances in studying black holes and neutron stars. The observatory’s high-resolution images have revealed the structure of accretion disks and relativistic jets emitted by supermassive black holes at the centers of galaxies. These observations have provided crucial data on the growth and behavior of black holes, as well as their influence on galaxy formation and evolution.
In addition, Chandra has been instrumental in mapping the hot gas in galaxy clusters, the largest structures in the universe. By observing the X-ray emissions from the hot intracluster medium, Chandra has helped determine the mass and distribution of dark matter in these clusters and provided insights into the large-scale structure of the cosmos.
The observatory has also contributed to the study of cosmic X-ray sources such as pulsars and X-ray binaries. Chandra’s ability to detect faint and distant X-ray sources has expanded our knowledge of these exotic objects, revealing details about their magnetic fields, spin rates, and interactions with their surroundings.
Spitzer Space Telescope: Infrared Insights
Launch and Mission of Spitzer
The Spitzer Space Telescope was launched on August 25, 2003, aboard a Delta II rocket from Cape Canaveral Air Force Station. Named after the American astrophysicist Lyman Spitzer, who pioneered the concept of space-based astronomy, Spitzer was designed to observe the universe in infrared wavelengths, which are crucial for studying celestial objects obscured by dust or too cool to emit visible light. The mission was managed by NASA’s Jet Propulsion Laboratory (JPL) and was part of NASA’s Great Observatories program, which also included the Hubble Space Telescope, the Chandra X-ray Observatory, and the Compton Gamma Ray Observatory.
Spitzer’s primary mission was to investigate the formation and evolution of galaxies, stars, and planetary systems. Its orbit around the Sun, trailing Earth, allowed it to remain in a stable thermal environment away from Earth’s heat and infrared emissions. This position enabled Spitzer to observe faint and distant objects with unprecedented sensitivity and clarity. The telescope was equipped with three main instruments: the Infrared Array Camera (IRAC), the Infrared Spectrograph (IRS), and the Multiband Imaging Photometer (MIPS), each designed to capture and analyze different aspects of the infrared spectrum.
Innovations in Infrared Observation
Spitzer’s design incorporated several innovative technologies to optimize its infrared observations. One of the key innovations was its use of a cryogenic cooling system to maintain its instruments at extremely low temperatures. Unlike other space telescopes, Spitzer was equipped with a large, liquid-helium-cooled cryostat that kept its detectors at temperatures just above absolute zero. This cooling system was essential for minimizing the thermal noise that could otherwise interfere with the detection of faint infrared signals from distant objects.
The telescope’s instruments were designed to cover a broad range of infrared wavelengths, from 3 to 180 micrometers. The Infrared Array Camera (IRAC) provided high-resolution images in the mid-infrared spectrum, allowing detailed studies of star formation regions and distant galaxies. The Infrared Spectrograph (IRS) offered spectroscopy capabilities to analyze the chemical compositions and physical conditions of celestial objects. The Multiband Imaging Photometer (MIPS) extended Spitzer’s range into the far-infrared spectrum, enabling observations of cooler and more distant objects, such as the earliest galaxies and the formation of planetary systems.
Additionally, Spitzer’s design included a sunshield to protect its instruments from the Sun’s heat and to maintain the telescope’s optimal thermal environment. This innovative feature ensured that Spitzer could operate efficiently for an extended period, allowing it to conduct long-term studies of various astronomical phenomena.
Key Discoveries from Spitzer
Spitzer made a multitude of groundbreaking discoveries during its mission, significantly advancing our understanding of the universe. One of its major achievements was the detailed study of star formation. Spitzer’s observations of dense molecular clouds and protostellar systems provided insights into the early stages of star formation, revealing the complex processes involved in the birth of stars and planetary systems. Its infrared imaging allowed scientists to peer through dust clouds that obscure visible light, offering a clearer view of these formative regions.
The telescope also made significant contributions to our understanding of galaxy formation and evolution. Spitzer’s observations of distant galaxies helped determine their age, size, and composition, providing evidence for the growth and development of galaxies over cosmic time. One notable discovery was the identification of some of the earliest galaxies formed shortly after the Big Bang, shedding light on the conditions of the early universe and the formation of the first cosmic structures.
In planetary science, Spitzer’s observations of the outer planets in our solar system, including Saturn and its moons, provided new insights into their atmospheres and ring systems. The telescope also made notable discoveries in the study of exoplanets. Spitzer’s data helped identify and characterize exoplanet atmospheres, including the detection of potential water vapor and other atmospheric components in some of these distant worlds.
Another significant contribution of Spitzer was its role in studying the distribution of interstellar dust and the structure of the Milky Way galaxy. The telescope’s far-infrared observations mapped the distribution of dust and gas within our galaxy, offering a better understanding of the processes shaping its structure and evolution.
Kepler Space Telescope: Hunting for Exoplanets
Kepler’s Mission and Objectives
The Kepler Space Telescope, launched on March 7, 2009, was a mission designed by NASA to search for Earth-like planets orbiting other stars. Named after the astronomer Johannes Kepler, whose laws of planetary motion laid the foundation for modern astronomy, the Kepler mission aimed to explore the frequency of planets within the habitable zones of their stars—regions where conditions might support liquid water and potentially life. The primary goal was to identify and characterize these planets, particularly those that are similar in size and composition to Earth.
Kepler’s primary scientific objective was to conduct a survey of a specific portion of the Milky Way galaxy, focusing on a field of view in the constellation Cygnus. The telescope used a photometer to continuously monitor the brightness of over 150,000 stars, looking for periodic dips in light intensity caused by planets transiting—or passing in front of—these stars. By analyzing these transits, Kepler was able to infer the presence of planets, their sizes, and their orbital characteristics.
The mission also aimed to provide statistical data on the frequency and distribution of planets, especially those located in the habitable zones of their stars. This information was crucial for understanding the potential for life elsewhere in the galaxy and assessing the prevalence of planetary systems similar to our own.
Breakthroughs in Exoplanet Detection
Kepler’s mission led to a series of groundbreaking discoveries in exoplanet detection. One of its most significant achievements was the identification of thousands of exoplanets, many of which were in the habitable zones of their stars. Kepler’s precise measurements allowed scientists to detect planets as small as Mars and determine their sizes and orbital periods with high accuracy.
A notable breakthrough was the discovery of a diverse range of exoplanetary systems, including the detection of multi-planet systems where several planets orbit the same star. This finding demonstrated that planetary systems can be complex and varied, and it provided valuable insights into the formation and evolution of such systems.
One of Kepler’s most famous discoveries was the identification of Kepler-186f, the first Earth-sized exoplanet found in the habitable zone of its star. This planet’s location raised the possibility that conditions on its surface could be suitable for liquid water. The detection of such potentially habitable planets sparked excitement about the potential for life beyond our solar system and influenced subsequent missions focused on finding and characterizing Earth-like worlds.
Kepler also observed planets with unusual properties, such as those with highly eccentric orbits or those that are significantly larger than Earth. These discoveries expanded our understanding of the variety of planetary systems in the galaxy and challenged existing theories about planet formation and orbital dynamics.
Impact on Understanding Planetary Systems
The Kepler mission profoundly impacted our understanding of planetary systems by revealing the vast diversity and prevalence of exoplanets. Prior to Kepler, only a few exoplanets had been discovered, most of which were large gas giants orbiting very close to their parent stars. Kepler’s extensive survey demonstrated that planets are common and that a wide range of planetary types exist, from rocky, Earth-sized planets to gas giants and ice giants.
Kepler’s data showed that many stars have planetary systems, and it provided statistical estimates for the occurrence of planets in the habitable zones of their stars. This information has been crucial for understanding the potential for life in the galaxy and for guiding the search for habitable planets. Kepler’s findings also highlighted that planets can form in a variety of environments, including those with high levels of stellar radiation or in binary star systems.
The mission’s discoveries have influenced follow-up observations and missions, such as the James Webb Space Telescope and various ground-based observatories, which aim to further investigate the properties of exoplanets and their atmospheres. Kepler’s data have also provided a foundation for studying the composition and structure of planetary atmospheres, particularly in the context of characterizing potential biosignatures.
James Webb Space Telescope: The Next Frontier
Overview of the James Webb Telescope
The James Webb Space Telescope (JWST) is the most advanced space telescope ever built and is considered the successor to the Hubble Space Telescope. Developed as an international collaboration between NASA, the European Space Agency (ESA), and the Canadian Space Agency (CSA), JWST was launched on December 25, 2021. Named after James E. Webb, a key figure in the Apollo program and a proponent of space science, the telescope is designed to investigate a wide range of astronomical phenomena, from the formation of stars and galaxies to the characteristics of exoplanets and their atmospheres.
JWST operates primarily in the infrared spectrum, allowing it to peer through cosmic dust clouds and observe distant objects that are too faint or obscured for other telescopes. Positioned at the second Lagrange point (L2), approximately 1.5 million kilometers (about 930,000 miles) from Earth, JWST benefits from a stable thermal environment and minimal interference from Earth’s atmosphere. This strategic location, combined with its advanced instruments, positions JWST to provide unprecedented views of the universe.
Technological Innovations and Capabilities
JWST is equipped with several groundbreaking technological innovations that enhance its observational capabilities. One of the most notable features is its large primary mirror, which spans 6.5 meters (21.3 feet) in diameter. The mirror is composed of 18 hexagonal segments made of beryllium, each coated with a thin layer of gold to optimize its reflection of infrared light. The large size of the mirror allows JWST to collect more light and observe fainter objects with greater clarity than previous space telescopes.
Another key innovation is the telescope’s advanced infrared instruments. JWST is equipped with four main scientific instruments: the Near Infrared Camera (NIRCam), the Near Infrared Spectrograph (NIRSpec), the Mid-Infrared Instrument (MIRI), and the Fine Guidance Sensor/Near Infrared Imager and Slitless Spectrograph (FGS/NIRISS). Each instrument is designed to detect different wavelengths of infrared light, enabling a wide range of scientific investigations.
The telescope’s sunshield is another significant technological advancement. The sunshield, which is about the size of a tennis court when fully deployed, protects the telescope from the Sun’s heat and provides a stable, cold environment for its instruments. The sunshield’s multi-layer design and precise deployment mechanisms ensure that JWST maintains optimal operating temperatures and minimizes thermal interference.
JWST’s ability to conduct precise observations is further enhanced by its high-precision attitude control system, which keeps the telescope accurately pointed at its targets. This capability is crucial for obtaining high-resolution images and spectra of distant and faint astronomical objects.
Expected Contributions to Astronomy
The James Webb Space Telescope is anticipated to make significant contributions across various fields of astronomy. One of its primary goals is to investigate the formation and evolution of the earliest galaxies. By observing the universe’s first galaxies and their formation processes, JWST is expected to provide insights into the conditions that led to the development of the cosmic structures we see today.
JWST will also enhance our understanding of star formation and the birth of planetary systems. Its infrared observations will allow scientists to study dense molecular clouds and protoplanetary disks with unprecedented detail, shedding light on the processes that lead to star and planet formation.
In the realm of exoplanet science, JWST is poised to revolutionize our knowledge of exoplanet atmospheres. Its advanced instruments will enable detailed analysis of the composition and structure of exoplanet atmospheres, including the potential detection of biosignatures and other markers of habitability. This capability will significantly advance our search for extraterrestrial life and our understanding of planetary environments.
Fermi Gamma-ray Space Telescope: Exploring the High-Energy Universe
Mission and Goals of Fermi
The Fermi Gamma-ray Space Telescope, launched on June 11, 2008, is a key observatory in the field of high-energy astrophysics. Named after the physicist Enrico Fermi, known for his work on nuclear reactions and cosmic rays, the mission is led by NASA in collaboration with the U.S. Department of Energy, the Italian Space Agency (ASI), and other international partners. The primary mission of Fermi is to explore the universe in gamma rays, the highest-energy form of electromagnetic radiation.
Fermi’s goals include studying the most energetic and dynamic phenomena in the universe, such as gamma-ray bursts, pulsars, supernova remnants, and active galactic nuclei. The telescope is designed to detect and analyze gamma rays from these sources, providing insights into their physical processes and the extreme environments in which they occur. By observing gamma rays, which can penetrate dense cosmic regions that block other forms of light, Fermi aims to uncover new aspects of high-energy astrophysics and improve our understanding of fundamental cosmic processes.
The mission also seeks to map the gamma-ray sky, creating a comprehensive catalog of gamma-ray sources and their properties. This catalog will help scientists study the distribution of high-energy phenomena across the universe and investigate their origins and interactions. Additionally, Fermi is designed to address key questions about the nature of dark matter by searching for potential signals from dark matter annihilations or decays.
Discoveries in Gamma-ray Astronomy
Since its launch, the Fermi Gamma-ray Space Telescope has made a multitude of significant discoveries in gamma-ray astronomy, transforming our understanding of the high-energy universe. One of its major achievements has been the detection and characterization of numerous gamma-ray sources across the sky. Fermi’s Large Area Telescope (LAT), the primary instrument onboard, has identified a wide range of gamma-ray sources, including pulsars, active galactic nuclei (AGN), and gamma-ray bursts (GRBs).
One of Fermi’s notable discoveries is the identification of thousands of new gamma-ray sources, many of which were previously unknown. The LAT has mapped the gamma-ray sky with unprecedented sensitivity and resolution, revealing previously hidden cosmic phenomena and providing new insights into their nature. The identification of these sources has led to a better understanding of their properties, such as their emission mechanisms and their role in the broader cosmic landscape.
Fermi has also provided valuable information about gamma-ray bursts, some of the most powerful explosions in the universe. The telescope’s observations have revealed detailed information about the afterglows of these bursts, allowing scientists to study their energy release and the processes driving these explosive events. Fermi’s data have contributed to the understanding of the various types of gamma-ray bursts and their implications for stellar evolution and cosmic explosions.
Another significant contribution of Fermi is its study of pulsars, highly magnetized, rotating neutron stars that emit beams of electromagnetic radiation. Fermi has detected gamma-ray emissions from many pulsars, providing insights into their magnetic fields, rotational dynamics, and particle acceleration processes. The telescope’s observations have helped refine models of pulsar behavior and improve our understanding of their role in the cosmic ecosystem.
The Fermi mission has also made advances in the study of active galactic nuclei, regions surrounding supermassive black holes at the centers of galaxies. Fermi’s observations have shed light on the high-energy processes occurring in these regions, including the acceleration of particles to near-light speeds and the production of powerful gamma-ray jets. These findings have enhanced our understanding of the mechanisms powering AGNs and their impact on galaxy evolution.
Wide-field Infrared Survey Explorer (WISE)
WISE Mission Overview
The Wide-field Infrared Survey Explorer (WISE) was a NASA mission launched on December 14, 2009, with the goal of surveying the entire sky in infrared wavelengths. The mission was designed to conduct a comprehensive, all-sky survey to detect and catalog a wide range of celestial objects that emit infrared radiation. WISE was equipped with a 40-centimeter (16-inch) telescope and four infrared detectors that covered the wavelength range from 3.4 to 22 micrometers.
WISE’s primary mission objectives were to explore the early universe, identify and study asteroids and comets in our solar system, and detect distant galaxies and star-forming regions. The telescope’s wide-field imaging capability allowed it to capture detailed, large-scale views of the sky, providing a wealth of data on various astronomical phenomena.
The mission consisted of two main phases. The first phase, known as the “Cryogenic Survey,” lasted from January 2010 to August 2011, during which WISE’s infrared detectors were cooled by a cryogenic system to maintain optimal performance. The second phase, the “Post-Cryogenic Survey,” began when the cryogen ran out, and WISE continued to operate with reduced capabilities until February 2011, focusing primarily on the detection of asteroids and comets.
Contributions to Infrared Astronomy
The WISE mission made several significant contributions to the field of infrared astronomy. One of its major achievements was the creation of the first comprehensive all-sky infrared survey. The extensive data collected by WISE provided a detailed map of the sky, revealing a wide range of celestial objects that were previously obscured by dust or too faint to be detected in visible light.
WISE’s survey greatly expanded our knowledge of near-Earth objects, including asteroids and comets. The mission identified thousands of new asteroids and comets, many of which were in the inner regions of the solar system. By tracking their orbits and physical properties, WISE contributed to our understanding of the composition and dynamics of these small bodies.
In the realm of star formation, WISE’s infrared observations revealed numerous star-forming regions that were hidden from view in visible light. The mission detected vast molecular clouds and young stellar objects, providing valuable insights into the processes that lead to star formation and the conditions within these formative environments.
The WISE mission also made notable discoveries in the study of brown dwarfs, which are substellar objects that are too cool to sustain hydrogen fusion but warmer than typical planets. WISE identified a large number of these objects, including some of the coldest known brown dwarfs. These discoveries have helped improve our understanding of the lower end of the stellar mass spectrum and the formation of substellar objects.
Transiting Exoplanet Survey Satellite (TESS)
Mission and Design of TESS
The Transiting Exoplanet Survey Satellite (TESS) is a NASA mission launched on April 18, 2018, designed to search for exoplanets, particularly those that are close to Earth and potentially habitable. TESS aims to build on the legacy of the Kepler Space Telescope by providing a more comprehensive survey of the sky and discovering a diverse range of exoplanets around bright, nearby stars.
TESS is equipped with four wide-field cameras that cover a large portion of the sky. The satellite’s primary mission is to monitor the brightness of thousands of stars in search of periodic dips in their light curves, which are indicative of planets transiting—or passing in front of—their host stars. By observing these transits, TESS can detect exoplanets and measure their sizes and orbital periods.
The spacecraft’s design includes a highly efficient system for data collection and transmission. TESS uses a series of planned observational sectors, each covering a large field of view for 27 days. The mission is divided into two primary phases: the initial mission phase, which focuses on a 2-year survey of the entire sky, and the extended mission phase, which continues to observe selected regions and conduct follow-up observations.
Impact on Exoplanet Research
TESS has significantly impacted exoplanet research by expanding the catalog of known exoplanets and providing valuable data on their characteristics. One of the mission’s major achievements is the discovery of thousands of exoplanet candidates around bright, nearby stars, which are ideal targets for follow-up studies. TESS’s observations have led to the identification of many new exoplanets, including those that are Earth-sized or smaller, which are particularly intriguing for the study of planetary habitability.
The mission has also contributed to the identification of a diverse range of exoplanetary systems. By observing stars in different regions of the sky, TESS has discovered planetary systems with a variety of configurations, including multi-planet systems and those with planets in unusual orbits. These discoveries provide new insights into the formation and evolution of planetary systems.
TESS’s observations are especially valuable for studying exoplanets that are close to Earth. The mission’s focus on bright stars allows for more detailed follow-up observations using ground-based telescopes and other space observatories. This has enabled scientists to characterize the atmospheres of several exoplanets, study their compositions, and search for potential biosignatures.
The data from TESS has also been instrumental in guiding subsequent missions and research efforts. For example, TESS’s discoveries have informed the selection of targets for the James Webb Space Telescope (JWST) and other observatories, which will conduct more detailed investigations of exoplanets and their atmospheres.
NuSTAR: Probing the High-Energy X-ray Universe
Overview and Mission of NuSTAR
The Nuclear Spectroscopic Telescope Array (NuSTAR) is a NASA mission designed to observe the universe in high-energy X-rays. Launched on June 13, 2012, NuSTAR is specifically tasked with studying the most energetic and dynamic phenomena in the cosmos, including black holes, neutron stars, and supernova remnants. The mission aims to enhance our understanding of these high-energy processes by providing detailed observations of X-ray emissions from the universe’s most extreme environments.
NuSTAR’s primary instrument is its pair of X-ray telescopes, which are equipped with state-of-the-art technology to focus X-rays with high precision. Unlike traditional X-ray telescopes that use grazing incidence optics, NuSTAR employs a novel design with nested, conical mirrors. This design allows the telescope to capture high-energy X-rays in the range of 3 to 79 kiloelectron volts (keV), which are otherwise absorbed by Earth’s atmosphere and cannot be observed from the ground.
The mission’s primary goals include investigating the structure and behavior of black holes, studying the remnants of supernova explosions, and exploring the high-energy processes occurring in active galactic nuclei (AGN) and pulsars. NuSTAR also aims to address fundamental questions about the origin and acceleration of cosmic rays and the formation of massive black holes in the early universe.
Significant Discoveries and Contributions
NuSTAR has made several significant contributions to high-energy astrophysics since its launch. One of its most notable achievements is the detailed imaging and analysis of black hole systems. The telescope has provided unprecedented observations of the regions surrounding black holes, including the accretion disks and relativistic jets. These observations have improved our understanding of how black holes grow and interact with their environment, offering new insights into the physics of these enigmatic objects.
The mission has also advanced our knowledge of neutron stars, particularly through its observations of pulsars and magnetars. NuSTAR’s high-resolution X-ray imaging has revealed intricate details about the surfaces and magnetic fields of these neutron stars. The data has provided valuable information about the extreme conditions in which neutron stars exist and has helped refine models of their interior structures and magnetic environments.
NuSTAR’s observations of supernova remnants have shed light on the remnants of massive stellar explosions. By studying the X-ray emission from these remnants, NuSTAR has provided new insights into the distribution of elements produced during supernovae and the dynamics of the explosion. The mission has also helped identify regions of high-energy particles and magnetic fields within these remnants, contributing to our understanding of the processes that shape the evolution of supernova remnants.
In the realm of active galactic nuclei, NuSTAR has investigated the high-energy emission from AGNs and their central supermassive black holes. The telescope’s observations have revealed the presence of highly energetic X-ray emission from the regions close to these black holes, providing clues about the physical processes occurring in the innermost regions of AGNs and the impact of these processes on galaxy evolution.
Additionally, NuSTAR has contributed to the study of cosmic rays by observing the high-energy X-ray emission from sources believed to be associated with cosmic ray acceleration. These observations have helped scientists understand the mechanisms behind cosmic ray production and acceleration in various astrophysical environments.
Techniques and Technologies
Advanced Imaging Techniques
Advanced imaging techniques are crucial for observing and analyzing astronomical phenomena, particularly those that involve high-energy processes or faint objects. In modern astronomy, these techniques enable scientists to capture detailed and high-resolution images of celestial objects across various wavelengths of light, including visible, infrared, and X-ray. One key advancement in imaging is the use of space-based telescopes, which avoid the distortions and limitations imposed by Earth’s atmosphere.
For instance, the use of high-resolution optics and innovative mirror designs, such as those employed by the Hubble Space Telescope and the James Webb Space Telescope, allows astronomers to obtain sharp and clear images of distant galaxies, star clusters, and nebulae. Techniques such as adaptive optics, which correct for atmospheric turbulence in ground-based observations, further enhance image quality by compensating for the effects of air movement.
In high-energy astronomy, specialized imaging techniques are used to detect X-rays and gamma rays. Instruments like the Nuclear Spectroscopic Telescope Array (NuSTAR) employ nested conical mirrors to focus high-energy X-rays, while gamma-ray telescopes use arrays of scintillators or detectors to capture gamma-ray bursts. These advanced techniques allow astronomers to study the most energetic and dynamic processes in the universe, such as black holes, supernovae, and gamma-ray bursts, with unprecedented detail.
Spectroscopy and its Importance
Spectroscopy is a fundamental tool in astronomy used to analyze the light emitted, absorbed, or scattered by celestial objects. By dispersing light into its component colors or wavelengths, spectroscopy provides critical information about the physical properties of astronomical objects, including their composition, temperature, density, and motion.
One of the primary applications of spectroscopy is to determine the chemical composition of stars, planets, and galaxies. By examining the absorption and emission lines in a spectrum, astronomers can identify the specific elements and molecules present in an object. This information is crucial for understanding the formation and evolution of celestial bodies, as well as the processes occurring within them.
Spectroscopy also plays a key role in measuring the redshift or blueshift of light from distant objects. This shift in the wavelength of light reveals the relative motion of an object with respect to Earth, providing insights into the expansion of the universe and the dynamics of galaxies and clusters. Observations of spectral lines can also help identify the presence of exoplanets by detecting the effects of their atmospheres on the light from their host stars.
In addition, spectroscopy is used to study the physical conditions in stellar and planetary atmospheres. By analyzing the spectral lines associated with various ionization states and molecular transitions, astronomers can infer properties such as temperature, pressure, and magnetic fields. This information is essential for understanding the environment around stars and planets, including the potential habitability of exoplanets.
Data Processing and Analysis
Data processing and analysis are critical components of modern astronomical research, enabling scientists to interpret complex datasets and extract meaningful information from observations. The process begins with the collection of raw data from telescopes and instruments, which is then subjected to a series of calibration and correction procedures to account for instrumental effects, noise, and other sources of error.
The first step in data processing involves calibrating the raw data to correct for systematic errors and instrumental biases. This includes tasks such as dark current subtraction, flat-field correction, and alignment of images. Once calibrated, the data is processed to create scientifically useful products, such as images, spectra, and light curves.
Advanced data analysis techniques are employed to interpret these products and extract scientific information. For imaging data, this may involve techniques such as image stacking, deconvolution, and feature extraction to enhance the visibility of faint or distant objects. For spectroscopic data, methods such as line fitting, continuum subtraction, and emission line analysis are used to identify and quantify spectral features.
Scientific Contributions and Discoveries
Understanding Star Formation and Evolution
Star formation is a fundamental process in astronomy, involving the birth, life, and death of stars. This process begins within vast clouds of gas and dust in space, known as molecular clouds. These clouds, primarily composed of hydrogen and helium, collapse under their own gravity, leading to the formation of dense regions called protostars. As these protostars continue to accumulate mass, they increase in temperature and pressure until nuclear fusion ignites in their cores, marking the birth of a new star.
The study of star formation involves observing these processes in various stages using different wavelengths of light. Infrared observations, for example, are particularly useful for peering through the dense dust clouds that often obscure young stars. Telescopes like the James Webb Space Telescope (JWST) are designed to detect these infrared emissions, providing insights into the early stages of star formation.
As stars evolve, they pass through various stages depending on their mass. Low-mass stars, like our Sun, enter a stable phase known as the main sequence, where they spend most of their lives fusing hydrogen into helium. Once the hydrogen is exhausted, these stars expand into red giants before shedding their outer layers and leaving behind a dense core known as a white dwarf.
Massive stars, on the other hand, undergo more dramatic changes. They fuse heavier elements in their cores and eventually explode in supernovae. This explosion disperses heavy elements into space and can lead to the formation of neutron stars or black holes. Understanding these evolutionary pathways helps astronomers piece together the lifecycle of stars and their impact on the galaxy, including the enrichment of the interstellar medium with heavy elements.
Exploration of Black Holes and Neutron Stars
Black holes and neutron stars are among the most extreme and fascinating objects in the universe. Black holes, formed from the remnants of massive stars after supernova explosions, have gravitational fields so strong that nothing, not even light, can escape them. They are characterized by their event horizon, the boundary beyond which escape is impossible, and singularity, a point of infinite density at the center.
The study of black holes involves detecting their effects on nearby matter. For instance, the observation of X-rays emitted from accretion disks—disks of matter spiraling into a black hole—provides indirect evidence of their presence. The Event Horizon Telescope (EHT), which captured the first image of a black hole’s event horizon in the galaxy M87, represents a significant milestone in understanding these enigmatic objects.
Neutron stars are remnants of massive stars that have exploded as supernovae. These incredibly dense objects are composed almost entirely of neutrons and have masses comparable to the Sun but radii of only about 10 kilometers (6 miles). Neutron stars are known for their extreme gravitational fields and rapid rotation, which can create powerful beams of electromagnetic radiation observed as pulsars.
The study of neutron stars involves analyzing their emission across various wavelengths, including radio, X-ray, and gamma rays. This multi-wavelength approach provides insights into their magnetic fields, rotation rates, and the physical conditions on their surfaces. Neutron stars also offer a natural laboratory for studying fundamental physics under extreme conditions, including the behavior of matter at nuclear densities and the nature of gravity.
Mapping the Universe’s Structure
Mapping the universe’s structure involves understanding the large-scale distribution of galaxies, clusters, and cosmic voids. This mapping helps astronomers study the formation and evolution of the cosmic web—a vast network of interconnected filaments and voids that constitutes the large-scale structure of the universe.
One of the key techniques for mapping the universe is galaxy redshift surveys. By measuring the redshift of galaxies—how their light is stretched to longer wavelengths as they move away from us—astronomers can determine their distances and map their distribution across cosmic time. Surveys like the Sloan Digital Sky Survey (SDSS) have provided detailed maps of millions of galaxies, revealing the intricate structure of the cosmic web.
Another important tool is gravitational lensing, which occurs when the gravitational field of a massive object, such as a galaxy or cluster, bends the light from objects behind it. By studying these distortions, astronomers can map the distribution of dark matter, an invisible component that influences the large-scale structure of the universe.
Impact on Cosmology
Big Bang Theory and Cosmic Microwave Background
The Big Bang Theory is the prevailing cosmological model that describes the origin and evolution of the universe. According to this theory, the universe began as an extremely hot and dense point approximately 13.8 billion years ago. It then expanded rapidly in a process known as cosmic inflation, cooling as it expanded and leading to the formation of matter, galaxies, and other cosmic structures over time.
One of the key pieces of evidence supporting the Big Bang Theory is the Cosmic Microwave Background (CMB) radiation. The CMB is the remnant heat from the initial explosion and is present throughout the universe as a faint, uniform glow. Detected in 1965 by Arno Penzias and Robert Wilson, this radiation provides a snapshot of the universe when it was just 380,000 years old. At that time, the universe had cooled enough for protons and electrons to combine into hydrogen atoms, allowing light to travel freely for the first time.
The CMB is remarkably uniform but contains small fluctuations in temperature and density. These fluctuations represent the primordial density variations that eventually led to the formation of galaxies and other structures. Detailed measurements of the CMB, particularly by missions such as the Planck satellite and the Wilkinson Microwave Anisotropy Probe (WMAP), have provided crucial insights into the early universe’s conditions and helped refine models of cosmic evolution.
Dark Matter and Dark Energy Research
Dark matter and dark energy are two of the most significant and enigmatic components of the universe. Dark matter is a form of matter that does not emit, absorb, or reflect light, making it invisible to current telescopes. However, its presence is inferred from its gravitational effects on visible matter. Observations of galaxy rotation curves, where the outer regions of galaxies rotate faster than expected based on visible matter alone, suggest the existence of dark matter. It is thought to make up about 27% of the universe’s mass-energy content.
Efforts to understand dark matter involve both astronomical observations and experimental physics. Observatories search for the effects of dark matter in large-scale cosmic structures, while experiments attempt to directly detect dark matter particles or produce them in particle accelerators. Despite extensive research, the exact nature of dark matter remains one of the biggest mysteries in modern physics.
Dark energy, on the other hand, is a mysterious form of energy thought to be responsible for the accelerated expansion of the universe. Discovered in the late 1990s through observations of distant supernovae, dark energy is estimated to constitute about 68% of the universe’s energy content. It exerts a repulsive force that counteracts gravity and causes the expansion of the universe to accelerate.
Research into dark energy involves studying its effects on cosmic expansion and structure formation. Observations of supernovae, galaxy clusters, and the large-scale structure of the universe provide clues about dark energy’s properties. Additionally, experiments like the Dark Energy Survey (DES) and the upcoming Euclid mission aim to map the distribution of dark energy and improve our understanding of its role in the universe’s evolution.
Expansion of the Universe
The expansion of the universe is a fundamental aspect of cosmology, describing how the universe has grown and continues to grow over time. This concept was first proposed by Edwin Hubble in the 1920s when he observed that distant galaxies are moving away from us, and the farther a galaxy is, the faster it appears to be receding. This observation led to the formulation of Hubble’s Law, which quantifies the rate of expansion.
The expansion of the universe is driven by the initial Big Bang and has been ongoing ever since. Initially, the expansion was rapid, driven by cosmic inflation, but it has gradually slowed due to the gravitational pull of matter. However, recent observations reveal that the expansion of the universe is now accelerating, a discovery attributed to dark energy.
The rate of expansion, known as the Hubble constant, is determined through various methods, including the observation of distant supernovae, the measurement of galaxy distributions, and the study of the CMB. The value of the Hubble constant provides crucial information about the size, age, and future of the universe.
Understanding the expansion of the universe also involves studying the large-scale structure and distribution of galaxies, as well as the role of dark matter and dark energy in shaping cosmic evolution. The interplay between these factors influences the growth of cosmic structures, the formation of galaxies, and the ultimate fate of the universe.
Collaboration and International Efforts
Joint Missions with Other Space Agencies
Joint missions between space agencies are collaborations that pool resources, expertise, and technology to achieve common scientific goals. These partnerships often involve multiple countries and organizations working together on space exploration, research, and observation projects. Such collaborations enhance the scientific value of missions and foster international cooperation in space exploration.
One notable example is the International Space Station (ISS), a collaborative effort involving NASA (United States), Roscosmos (Russia), ESA (European Space Agency), JAXA (Japan Aerospace Exploration Agency), and CSA (Canadian Space Agency). The ISS serves as a microgravity laboratory for scientific research across a range of disciplines, including astronomy, biology, and materials science. It demonstrates how joint missions can provide a platform for shared scientific endeavors and long-term space habitation.
Another prominent joint mission is the European Space Agency’s (ESA) involvement in NASA’s James Webb Space Telescope (JWST). ESA contributed the Ariane 5 rocket for the JWST’s launch and provided scientific and technical expertise throughout its development. The collaboration highlights the importance of pooling international resources to create advanced space observatories capable of exploring the universe’s most profound questions.
The collaboration on missions like the Mars rovers, with NASA and ESA contributing to the Mars Science Laboratory and ExoMars missions, showcases the benefits of sharing knowledge and technology. These joint efforts aim to explore Mars, search for signs of past life, and understand the planet’s geology and climate. By working together, space agencies can achieve more ambitious goals and advance our understanding of the solar system.
International Data Sharing and Collaboration
International data sharing and collaboration in space research are essential for maximizing the scientific impact of space missions and fostering global cooperation. By sharing data and findings, space agencies and research institutions can enhance their collective understanding of the universe and address complex scientific questions that require a broad range of expertise and resources.
Data sharing allows researchers to access and analyze data from a variety of missions and instruments, providing a more comprehensive view of celestial phenomena. For instance, the data collected by NASA’s Hubble Space Telescope is made available to the global scientific community, enabling researchers from around the world to conduct studies and publish findings. Similarly, data from the European Space Agency’s Gaia mission, which maps the positions and motions of stars, is shared internationally, facilitating collaborative research on stellar and galactic dynamics.
International collaborations also involve joint scientific publications and conferences, where researchers present and discuss their findings. These events foster the exchange of ideas and methodologies, leading to new insights and innovations. For example, the collaboration between space agencies and research institutions on the study of exoplanets involves sharing data from telescopes like Kepler, TESS, and JWST, which contributes to a global understanding of planetary systems and their potential for habitability.
Moreover, data sharing agreements and partnerships, such as those established through the International Space Science Institute (ISSI) and the Space Data Association (SDA), help coordinate and manage data access across international projects. These agreements ensure that data from space missions are available to researchers worldwide, promoting transparency and collaboration in scientific research.
Public Engagement and Education
Public Outreach Programs
Public outreach programs are essential for bridging the gap between the scientific community and the general public, fostering interest in space exploration, and enhancing scientific literacy. These programs aim to communicate complex scientific concepts in an accessible and engaging manner, making space science more relatable and understandable to people of all ages.
One example of a successful public outreach program is NASA’s “NASA Science” initiative, which provides a variety of resources including articles, videos, and interactive tools about ongoing missions and discoveries. Through its website and social media platforms, NASA engages with the public by sharing updates on missions, showcasing images and videos from space, and hosting live events such as rover landings and satellite launches.
Space agencies also organize public events such as open houses, where visitors can tour facilities, meet scientists, and learn about the latest research and technology. The European Space Agency (ESA) and the National Aeronautics and Space Administration (NASA) frequently hold such events to provide firsthand experiences of space missions and encourage public interest in space exploration.
Additionally, space-themed exhibitions and planetarium shows offer immersive experiences that allow visitors to explore the universe virtually. These programs often feature interactive displays, simulations, and educational presentations that bring space science to life, making it more engaging for audiences.
Educational Initiatives and Resources
Educational initiatives and resources play a crucial role in inspiring the next generation of scientists, engineers, and explorers. These programs provide students and educators with tools, materials, and opportunities to learn about space science and engineering in a hands-on and interactive manner.
One prominent educational initiative is NASA’s “STEM Engagement” program, which offers a range of resources for educators and students. These include lesson plans, classroom activities, and educational videos designed to align with curriculum standards. NASA also provides opportunities for students to participate in competitions and projects, such as the “Moon to Mars” challenge, which encourages students to design and build models related to lunar exploration.
Space agencies often collaborate with educational institutions and organizations to develop curriculum materials and teacher training programs. For example, ESA’s “Space in Schools” program offers educational resources and activities that help teachers integrate space science into their lessons, fostering students’ interest in STEM (Science, Technology, Engineering, and Mathematics) fields.
Furthermore, space agencies and research institutions frequently partner with museums, science centers, and planetariums to offer educational programs and workshops. These collaborations create interactive learning experiences that engage students and the public, promoting a deeper understanding of space science and its applications.
Impact on Popular Culture
Space exploration has had a profound impact on popular culture, influencing art, literature, film, and television. The fascination with space and its mysteries has inspired countless works that capture the imagination and reflect societal attitudes toward space exploration.
Science fiction, in particular, has been a significant vehicle for exploring themes related to space exploration. Films such as “2001: A Space Odyssey” and “Interstellar” have not only entertained audiences but also stimulated interest in space science and technology. These movies often incorporate scientific concepts and theories, sometimes even influencing public perceptions and expectations about space missions and technological advancements.
Television series like “Star Trek” and “The Expanse” have similarly shaped popular culture by presenting futuristic visions of space exploration and interstellar travel. These shows often highlight scientific principles and speculative technologies, contributing to public interest in space and space science.
In literature, space exploration has been a central theme in works by authors such as Arthur C. Clarke and Isaac Asimov. These books explore the possibilities of space travel, alien life, and the future of humanity, reflecting both scientific curiosity and imaginative speculation.
The impact of space exploration on popular culture is also evident in the arts, including music, visual arts, and fashion. For example, the iconic imagery of astronauts and spacecraft has influenced design aesthetics and artistic expression, while space-themed music and fashion often evoke themes of exploration and discovery.
Future Prospects and Missions
Upcoming NASA Space Telescopes
NASA’s upcoming space telescopes promise to advance our understanding of the universe by extending the capabilities of current instruments and exploring new scientific frontiers. These next-generation telescopes are designed to address key questions in astronomy and astrophysics, providing deeper insights into the cosmos.
One of the most anticipated upcoming telescopes is the Nancy Grace Roman Space Telescope (formerly known as WFIRST). Scheduled for launch in the mid-2020s, this telescope will carry a wide-field instrument capable of surveying large areas of the sky with high precision. Its primary scientific goals include investigating the nature of dark energy, studying the formation and evolution of galaxies, and detecting exoplanets through microlensing. The Roman Space Telescope’s expansive field of view will allow astronomers to explore cosmic phenomena on a much larger scale than current telescopes.
Another exciting project is the Habitable Exoplanet Observatory (HabEx), which is under consideration by NASA and is part of the decadal survey for astronomy and astrophysics. HabEx aims to directly image and characterize Earth-like exoplanets in the habitable zones of nearby stars. Its advanced coronagraph and starshade technology will enable detailed studies of exoplanet atmospheres, searching for signs of habitability and potentially life.
The Origins Space Telescope (OST) is another upcoming mission proposed to explore the universe’s earliest galaxies and star formation. OST is designed to operate in the far-infrared spectrum, providing unprecedented sensitivity and resolution to study the formation of stars and galaxies in the early universe. This telescope will address fundamental questions about the formation of cosmic structures and the origins of the first stars and galaxies.
Future Collaborations and Innovations
Future collaborations between NASA and international space agencies, as well as private organizations, are expected to drive innovative breakthroughs in space exploration and research. These partnerships will leverage shared resources, expertise, and technology to achieve ambitious scientific goals and explore new frontiers.
One prominent example of future collaboration is the Lunar Gateway, a planned space station that will orbit the Moon. The Lunar Gateway is a collaborative effort involving NASA, ESA, JAXA, and CSA, aimed at supporting long-term lunar exploration and serving as a staging point for missions to Mars. This international partnership will enable the development of new technologies, conduct scientific research, and test systems required for deep space exploration.
NASA’s Artemis program, which aims to return humans to the Moon and establish a sustainable presence, will also benefit from international and commercial partnerships. Collaborations with space agencies such as ESA, Roscosmos, and private companies like SpaceX and Blue Origin will facilitate the development and deployment of new lunar landers, habitats, and exploration technologies.
Innovations in space technology will continue to emerge from these collaborative efforts, including advancements in propulsion systems, space habitats, and robotic exploration. For example, international partnerships in the development of advanced propulsion technologies, such as nuclear thermal propulsion and ion drives, will enhance the capability to conduct deep space missions and explore distant planets.
Long-term Goals and Visions
NASA’s long-term goals and visions are centered around expanding human presence in space, exploring the solar system, and advancing our understanding of the universe. These goals include establishing a sustainable presence on the Moon, sending humans to Mars, and searching for signs of life beyond Earth.
A key component of NASA’s long-term vision is the Artemis program, which aims to return astronauts to the Moon by the mid-2020s and establish a sustainable lunar presence by the end of the decade. This program is designed to lay the groundwork for future exploration of Mars and other destinations, including the development of new technologies and the establishment of a lunar base that can support long-duration missions.
Another ambitious goal is the exploration of Mars, with plans to send humans to the Red Planet in the 2030s. NASA’s Mars exploration efforts include the development of advanced spacecraft, habitats, and life support systems to enable human missions. These efforts also involve studying Mars’ geology, climate, and potential for past or present life, as well as preparing for future missions that may involve sample return and long-term habitation.
In addition to planetary exploration, NASA’s long-term vision includes advancing our understanding of fundamental cosmic questions, such as the nature of dark matter and dark energy, the formation of galaxies and stars, and the search for extraterrestrial life. Future space telescopes, observatories, and missions will play a crucial role in addressing these questions and expanding our knowledge of the universe.
Challenges and Solutions
Technical and Engineering Challenges
Space telescopes face numerous technical and engineering challenges that must be addressed to ensure their successful operation and scientific output. These challenges range from the complexity of their design and construction to the harsh conditions of space.
One of the primary technical challenges is the deployment and calibration of sophisticated instruments in space. Space telescopes are equipped with highly sensitive detectors and optics that must be precisely aligned and calibrated to function correctly. For instance, the deployment of the James Webb Space Telescope’s sunshield and its segmented mirror required intricate engineering to ensure proper unfolding and alignment, crucial for its mission success.
Thermal control is another significant challenge. Space telescopes operate in extreme temperature environments, with temperatures varying widely between sunlight and shadow. Engineers must design and implement advanced thermal control systems to maintain the telescope’s components within their operational temperature range. This includes using insulation, heaters, and radiators to manage heat dissipation and prevent thermal-induced distortions of the telescope’s optics and instruments.
Additionally, space telescopes must withstand the space environment, including radiation and micrometeoroids. The harsh conditions of space require robust shielding and protective coatings to prevent damage to sensitive components. For instance, the Hubble Space Telescope’s protective coatings help shield its instruments from space radiation and micro-meteoroid impacts.
Communication with Earth also presents challenges. Space telescopes must transmit large volumes of data across vast distances, which requires efficient data transmission systems and reliable communication links. Engineers must balance data quality and transmission speed with the constraints of available bandwidth and signal strength.
Funding and Budget Considerations
Funding and budget considerations are crucial aspects of space telescope projects, influencing their scope, development timeline, and overall success. Developing and launching space telescopes involves substantial financial investment, with costs related to design, construction, testing, launch, and operations.
Securing funding for space telescopes involves competing for budget allocations within national space agencies and other funding bodies. Space missions often compete with other scientific, exploration, and defense priorities, which can impact their funding levels. For instance, large projects like the James Webb Space Telescope have faced budget overruns and delays due to the complexity and scale of the mission.
To manage costs, space agencies often implement rigorous project management practices, including detailed budgeting, cost estimation, and financial oversight. Effective project management helps mitigate risks and manage expenses while ensuring that the mission stays within budget. For example, the Hubble Space Telescope’s initial costs were offset by its extended mission life and the scientific returns it provided, which justified the investment.
Collaborative missions involving international partners or private industry can also impact funding strategies. Shared costs and resources among multiple space agencies or commercial entities can help distribute the financial burden and make ambitious projects more feasible. For example, the International Space Station (ISS) is a collaborative effort that spreads the costs and responsibilities among participating nations.
Policy and International Cooperation
Policy and international cooperation play vital roles in the success and management of space telescope missions. Space exploration often involves complex legal, diplomatic, and policy considerations, particularly when multiple countries or organizations are involved.
Space policy frameworks govern the planning, execution, and management of space missions, including space telescopes. National space agencies must navigate regulations related to space traffic management, space debris mitigation, and the use of space resources. These policies ensure that space missions are conducted safely and sustainably, minimizing risks to both operational satellites and future space activities.
International cooperation is essential for achieving large-scale space missions and scientific goals. Collaborations between space agencies, such as NASA, ESA, JAXA, and others, facilitate the sharing of expertise, resources, and technology. For example, the collaboration on the Hubble Space Telescope involved contributions from NASA and ESA, including scientific instruments and funding. Such partnerships enhance the scientific capabilities of missions and promote global engagement in space exploration.
International agreements, such as the Outer Space Treaty and the Agreement on the Rescue of Astronauts, provide a legal framework for cooperation and the peaceful use of outer space. These agreements outline principles for international collaboration, including the prohibition of weapons in space and the commitment to avoid harmful contamination of celestial bodies.
Summary
Recap of NASA’s Space Telescopes’ Achievements
NASA’s space telescopes have significantly advanced our understanding of the universe, providing groundbreaking insights across various fields of astronomy and astrophysics. These achievements have reshaped our knowledge of cosmic phenomena and deepened our comprehension of the universe’s origins and evolution.
The Hubble Space Telescope, launched in 1990, has been instrumental in numerous discoveries. Its high-resolution images have revealed the intricate structures of galaxies, nebulae, and star clusters. Hubble’s observations have led to the precise determination of the universe’s expansion rate, the discovery of exoplanets, and the identification of distant galaxies that contributed to our understanding of cosmic evolution. Notable achievements include the determination of the age of the universe and the observation of the Hubble Deep Field, which provided a glimpse into the universe’s early stages.
The Chandra X-ray Observatory, operational since 1999, has revolutionized our view of the universe in the X-ray spectrum. Chandra’s observations have unveiled the existence of black holes and neutron stars, provided insights into the structure of galaxy clusters, and illuminated the remnants of supernova explosions. Its high-resolution X-ray imaging has allowed scientists to study the most energetic and extreme cosmic phenomena with unprecedented detail.
The Spitzer Space Telescope, launched in 2003, has provided critical data on the universe in the infrared spectrum. Spitzer’s discoveries include the identification of distant galaxies, the observation of star formation processes within dust clouds, and the detailed study of exoplanet atmospheres. Its infrared observations have offered valuable insights into the early universe and the formation of planetary systems.
The Kepler Space Telescope, which began its mission in 2009, has been pivotal in the search for exoplanets. Kepler’s extensive data has led to the discovery of thousands of exoplanets, including those in the habitable zones of their stars. Its contributions have significantly advanced our understanding of planetary systems and the potential for life beyond Earth.
The James Webb Space Telescope (JWST), launched in 2021, is poised to continue this legacy. With its advanced infrared capabilities, JWST aims to investigate the formation of the first galaxies, the evolution of star systems, and the atmospheres of exoplanets, providing insights into the early universe and the conditions that might support life.
Future Outlook and Potential Discoveries
The future of space telescopes promises to be as transformative as their past achievements, with new missions and technologies poised to explore previously uncharted realms of the universe. As these advanced instruments come online, they will address some of the most profound questions in astronomy and astrophysics.
Upcoming missions like the Nancy Grace Roman Space Telescope are expected to provide deeper insights into the nature of dark energy and the formation of galaxies. Its wide-field imaging capabilities will enable large-scale surveys of the universe, potentially uncovering new aspects of cosmic structure and expansion.
The Habitable Exoplanet Observatory (HabEx) and the Origins Space Telescope (OST) will focus on characterizing exoplanets and studying the early universe. HabEx aims to directly image Earth-like exoplanets and analyze their atmospheres, which could reveal signs of habitability or even life. OST will offer unprecedented views of the universe’s first galaxies and star formation processes, shedding light on the conditions that led to the development of cosmic structures.
The Lunar Gateway, an international collaboration, will support lunar exploration and serve as a base for future missions to Mars. Its presence will facilitate long-term studies of the Moon, aid in the development of new space technologies, and act as a staging point for deeper space exploration.
As space telescopes continue to advance, they will likely uncover new phenomena and refine our understanding of known processes. The study of dark matter and dark energy, the search for life beyond Earth, and the exploration of the universe’s earliest epochs are all areas poised for significant breakthroughs.
FAQs
What Was the First NASA Space Telescope?
NASA’s first space telescope was the Orbiting Astronomical Observatory (OAO-2), launched on December 7, 1968. The OAO-2 was designed to observe the universe in ultraviolet light, which is blocked by Earth’s atmosphere and thus requires space-based instruments for detection. This pioneering mission marked a significant step in space-based astronomy, providing valuable data that paved the way for future observatories. The success of OAO-2 demonstrated the potential of space telescopes to explore cosmic phenomena beyond the reach of ground-based instruments, setting the stage for subsequent missions that would greatly expand our understanding of the universe.
How Do Space Telescopes Differ from Ground-Based Telescopes?
Space telescopes differ from ground-based telescopes primarily due to their location outside Earth’s atmosphere. This placement allows space telescopes to avoid the atmospheric distortion that can blur images and limit observational capabilities on Earth. By operating above the atmosphere, space telescopes can capture clearer and more detailed images across a wider range of wavelengths, including those that are blocked by the atmosphere, such as ultraviolet and X-rays. In contrast, ground-based telescopes must contend with atmospheric interference, weather conditions, and light pollution, which can affect their observational quality. While space telescopes provide superior clarity and access to additional wavelengths, ground-based telescopes benefit from lower costs and the ease of upgrades and maintenance.
What Are the Most Significant Discoveries Made by Hubble?
The Hubble Space Telescope has made several groundbreaking discoveries that have profoundly impacted our understanding of the universe. One of its most significant achievements is determining the rate of the universe’s expansion, which led to the confirmation of dark energy—a mysterious force accelerating the expansion. Hubble’s imaging capabilities also enabled the Hubble Deep Field, which revealed thousands of previously unseen galaxies and provided insights into the universe’s early stages. Additionally, Hubble has been crucial in detecting and characterizing exoplanets through observations of transits and has produced detailed images of cosmic phenomena such as the Pillars of Creation and the Crab Nebula, offering new perspectives on stellar and galactic processes.
How Has the James Webb Space Telescope Improved Upon Hubble?
The James Webb Space Telescope (JWST) enhances and extends the capabilities of the Hubble Space Telescope through several key improvements. Unlike Hubble, which operates primarily in visible and ultraviolet light, JWST is designed to observe the universe in infrared wavelengths. This shift allows JWST to peer through cosmic dust and observe objects that are too faint or distant for Hubble, such as the earliest galaxies and star-forming regions. JWST’s larger 6.5-meter primary mirror, compared to Hubble’s 2.4 meters, provides greater light-gathering power and resolution. Additionally, JWST’s advanced instruments and improved thermal stability, thanks to its large sunshield, enable it to capture more detailed and accurate data, advancing our knowledge of the universe in ways that Hubble could not.
What Is the Future of Space Telescopes?
The future of space telescopes promises continued advancements in our exploration of the cosmos, driven by new missions and technological innovations. Upcoming telescopes like the Nancy Grace Roman Space Telescope and the Habitable Exoplanet Observatory (HabEx) are set to enhance our understanding of dark energy, exoplanets, and cosmic structures. These future missions will leverage advanced technologies to study the universe in unprecedented detail, addressing fundamental questions about its formation and evolution. As international collaborations and private sector involvement increase, space telescopes will benefit from shared expertise and resources, enabling more ambitious projects. Innovations in telescope design and instrumentation will further expand observational capabilities, leading to new discoveries and deeper insights into the nature of the universe.